The Role of Exosomes in the Pathogenesis of Chemotherapy-induced Cardiotoxicity: A Diagnostic and Prognostic Approach
Exosome and Cardiotoxicity
DOI:
https://doi.org/10.31661/gmj.v12i.2534Keywords:
Exosome, Cardiotoxicity, Pathogenesis, Chemotherapy, DiagnosisAbstract
Exosomes are a subtype of natural extracellular vesicles that transfer a great number of biomolecules, released by almost all types of cells, and can be specifically taken up by recipient cells in order to maintain homeostasis and induce a proper response to stress or harsh environmental conditions. Since the heart is part of complex systems which involve various cell types, exosome-mediated communication plays a crucial role in the cardiovascular field. Given the extended studies on the molecular mechanisms mediated by exosome cargos, leading to cardiovascular diseases, we focus on the most important data that report the roles of proteins and microRNAs (miRNAs) transferred by exosomes and whether they possess cardiotoxicity or cardioprotection and also the potential of these biomolecules as therapeutic tools or diagnostic biomarkers.
References
Nagai H, Kim YH. Cancer prevention from the perspective of global cancer burden patterns. J Thorac Dis. 2017;9(3):448-51.
https://doi.org/10.21037/jtd.2017.02.75
Pai VB, Nahata MC. Cardiotoxicity of chemotherapeutic agents: incidence, treatment and prevention. Drug saf. 2000;22(4):263-302.
https://doi.org/10.2165/00002018-200022040-00002
Tai YL, Chen KC, Hsieh JT, Shen TL. Exosomes in cancer development and clinical applications. Cancer Sci. 2018;109(8):2364-74.
https://doi.org/10.1111/cas.13697
Soleimani P, Yadollahifarsani S, Motieian M, Chenarani Moghadam MS, Alimohammadi S, Khayyat A, Esmaeil Pour MA, Neshat S, Marashi NA, Mahmoodnia L, Masomi R. Cancer recurrence or aggravation following COVID-19 vaccination. J Nephropharmacol. 2023;12(2):e10593.
https://doi.org/10.34172/npj.2023.10593
Zhang Y, Liu Y, Liu H, Tang WH. Exosomes: biogenesis, biologic function and clinical potential. Cell Biosci. 2019;9(1):19.
https://doi.org/10.1186/s13578-019-0282-2
Xu MY, Ye ZS, Song XT, Huang RC. Differences in the cargos and functions of exosomes derived from six cardiac cell types: a systematic review. Stem cell res ther. 2019;10(1):194.
https://doi.org/10.1186/s13287-019-1297-7
Bellin G, Gardin C, Ferroni L, Chachques JC, Rogante M, Mitrečić D, et al. Exosome in Cardiovascular Diseases: A Complex World Full of Hope. Cells. 2019;8(2):166.
https://doi.org/10.3390/cells8020166
Yazdani M, Riahi Samani P, Mazdak H, Yazdani A. Rate of clinically significant prostate cancer on repeated biopsy after a diagnosis of atypical small acinar proliferation. Immunopathol Persa. 2023;9(1):e94.
https://doi.org/10.34172/ipp.2022.94
Mohammadtabar M, Fazeli A, Parsai N, Aboulfathiyarmohammadyar Z, Shafiei E, Khaledi M, Zaremoghadam E, Rahnama Sisakht A, Mohammadi S, Mardanparvar H. Association between SGLT2 (sodium-glucose cotransporter-2) inhibitors and bladder cancer in individuals with type 2 diabetes; a systematic review and meta-analysis. J Nephropathol. 2023;12(2):e21444.
https://doi.org/10.34172/jnp.2023.21444
Nasri H. The role of exosome in different types of rejection in kidney transplantation; a diagnostic and prognostic approach. J Parathyr Dis. 2020;8:e11166.
Vlassov AV, Magdaleno S, Setterquist R, Conrad R. Exosomes: current knowledge of their composition, biological functions, and diagnostic and therapeutic potentials. Biochim Biophys Acta. 2012;1820(7):940-8.
https://doi.org/10.1016/j.bbagen.2012.03.017
Fakhredini F, Mansouri E, Orazizadeh M. Isolation and characterization of exosomes derived from supernatant of rabbit kidney tubular cells culture. J Prev Epidemiol. 2022;7(2):e26161.
https://doi.org/10.34172/jpe.2022.26161
Haraszti RA, Didiot MC, Sapp E, Leszyk J, Shaffer SA, Rockwell HE, et al. High-resolution proteomic and lipidomic analysis of exosomes and microvesicles from different cell sources. J Extracell Vesicles. 2016;5:32570.
https://doi.org/10.3402/jev.v5.32570
Zheng B, Yin W-N, Suzuki T, Zhang X-H, Zhang Y, Song L-L, et al. Exosome-Mediated miR-155 Transfer from Smooth Muscle Cells to Endothelial Cells Induces Endothelial Injury and Promotes Atherosclerosis. Mol Ther. 2017;25(6):1279-94.
https://doi.org/10.1016/j.ymthe.2017.03.031
Tijsen AJ, Creemers EE, Moerland PD, Windt LJd, Wal ACvd, Kok WE, et al. MiR423-5p As a Circulating Biomarker for Heart Failure. Circ Res. 2010;106(6):1035-9.
https://doi.org/10.1161/CIRCRESAHA.110.218297
Mahmoodi Z, Salarzaei M, Sheikh M. Prosthetic vascular graft infection: A systematic review and meta-analysis on diagnostic accuracy of 18FDG PET/CT. Gen Thorac Cardiovasc Surg. 2022 Mar;70(3):219-229.
https://doi.org/10.1007/s11748-021-01682-6
Ji Q, Ji Y, Peng J, Zhou X, Chen X, Zhao H, et al. Increased Brain-Specific MiR-9 and MiR-124 in the Serum Exosomes of Acute Ischemic Stroke Patients. PLoS One. 2016;11(9):e0163645.
https://doi.org/10.1371/journal.pone.0163645
Kasner M, Gast M, Galuszka O, Stroux A, Rutschow S, Wang X, et al. Circulating exosomal microRNAs predict functional recovery after MitraClip repair of severe mitral regurgitation. Int J Cardiol. 2016;215:402-5.
https://doi.org/10.1016/j.ijcard.2016.04.018
Kanhai DA, de Kleijn DP, Kappelle LJ, Uiterwaal CS, van der Graaf Y, Pasterkamp G, et al. Extracellular vesicle protein levels are related to brain atrophy and cerebral white matter lesions in patients with manifest vascular disease: the SMART-MR study. BMJ open. 2014;4(1):e003824.
https://doi.org/10.1136/bmjopen-2013-003824
Tian C, Yang Y, Bai B, Wang S, Liu M, Sun RC, et al. Potential of exosomes as diagnostic biomarkers and therapeutic carriers for doxorubicin-induced cardiotoxicity. Int J Biol Sci. 2021;17(5):1328-38.
https://doi.org/10.7150/ijbs.58786
Gupta S, Knowlton AA. HSP60 trafficking in adult cardiac myocytes: role of the exosomal pathway. Am J Physiol Heart Circ Physiol. 2007;292(6):H3052-6.
https://doi.org/10.1152/ajpheart.01355.2006
Duan Y, Tang H, Mitchell-silbaugh K, Fang X, Han Z, Ouyang K. Heat Shock Protein 60 in Cardiovascular Physiology and Diseases. Front Mol Biosci. 2020;7:73.
https://doi.org/10.3389/fmolb.2020.00073
Sheykh M, Ostadrahimi P, Havasian MR, Rostami-Estabragh K, Mahmoodi Z. Evaluation of gender differences in the prevalence of coronary risk factors in patients with acute intractable syndrome hospitalized in CCU of Amir-Almomenin hospital, Zabol. Res J of Pharm and Tech. 2017;10(9):2883-6.
https://doi.org/10.5958/0974-360X.2017.00509.1
Hu X, Liao W, Teng L, Ma R, Li H. Circ_0001312 Silencing Suppresses Doxorubicin-Induced Cardiotoxicity via MiR-409-3p/HMGB1 Axis. Int Heart J. 2023;64(1):71-80.
https://doi.org/10.1536/ihj.22-379
Zadeh FJ, Ghasemi Y, Bagheri S, Maleknia M, Davari N, Rezaeeyan H. Do exosomes play role in cardiovascular disease development in hematological malignancy? Mol Biol Rep. 2020 Jul;47(7):5487-5493.
https://doi.org/10.1007/s11033-020-05453-z
St Paul A, Corbett CB, Okune R, Autieri MV. Angiotensin II, Hypercholesterolemia, and Vascular Smooth Muscle Cells: A Perfect Trio for Vascular Pathology. Int J Mol Sci. 2020;21(12):4525.
https://doi.org/10.3390/ijms21124525
Molkentin JD. Parsing good versus bad signaling pathways in the heart: role of calcineurin-nuclear factor of activated T-cells. Circ res. 2013;113(1):16-9.
https://doi.org/10.1161/CIRCRESAHA.113.301667
Pagiatakis C, Gordon J, Ehyai S, McDermott J. A novel RhoA/ROCK-CPI-17-MEF2C signaling pathway regulates vascular smooth muscle cell gene expression. J Biol Chem. 2012;287:8361-70.
https://doi.org/10.1074/jbc.M111.286203
Shahramian I, Tabrizian K, Ostadrahimi P, Afshari M, Soleymanifar M, Bazi A. Therapeutic effects of ursodeoxycholic acid in neonatal indirect hyperbilirubinemia: a randomized double-blind clinical trial. Archives of Anesthesiology and Critical Care. 2019 Jul 2;5(3):99-103.
https://doi.org/10.18502/aacc.v5i3.1211
Datta R, Bansal T, Rana S, Datta K, Datta Chaudhuri R, Chawla-Sarkar M, et al. Myocyte-Derived Hsp90 Modulates Collagen Upregulation via Biphasic Activation of STAT-3 in Fibroblasts during Cardiac Hypertrophy. Mol Cell Biol. 2017;37(6):e00611-16.
https://doi.org/10.1128/MCB.00611-16
Sato N, Yamamoto T, Sekine Y, Yumioka T, Junicho A, Fuse H, et al. Involvement of heat-shock protein 90 in the interleukin-6-mediated signaling pathway through STAT3. Biochem Biophys Res Commun. 2003;300(4):847-52.
https://doi.org/10.1016/S0006-291X(02)02941-8
Chen F, Chen D, Zhang Y, Jin L, Zhang H, Wan M, et al. Interleukin-6 deficiency attenuates angiotensin II-induced cardiac pathogenesis with increased myocyte hypertrophy. Biochem Biophys Res Commun. 2017;494(3):534-41.
https://doi.org/10.1016/j.bbrc.2017.10.119
Marunouchi T, Nakashima M, Ebitani S, Umezu S, Karasawa K, Yano E, et al. Hsp90 Inhibitor Attenuates the Development of Pathophysiological Cardiac Fibrosis in Mouse Hypertrophy via Suppression of the Calcineurin-NFAT and c-Raf-Erk Pathways. J Cardiovasc Pharmacol. 2021;77(6):822-9.
https://doi.org/10.1097/FJC.0000000000001017
Huang H, Weng H, Zhou H, Qu L. Attacking c-Myc: Targeted and Combined Therapies for Cancer. Curr Pharm Des. 2014;20:6543-54.
https://doi.org/10.2174/1381612820666140826153203
Mehrpisheh S, Mosayebi Z, Memarian A, Kadivar M, Nariman S, Ostadrahimi P, Dalili H. Evaluation of specificity and sensitivity of gastric aspirate shake test to predict surfactant deficiency in Iranian premature infants. Pregnancy Hypertens. 2015 Apr;5(2):182-6.
https://doi.org/10.1016/j.preghy.2015.01.006
Parichatikanond W, Luangmonkong T, Mangmool S, Kurose H. Therapeutic Targets for the Treatment of Cardiac Fibrosis and Cancer: Focusing on TGF-β Signaling. Front Cardiovasc Med. 2020;7:34.
https://doi.org/10.3389/fcvm.2020.00034
Yu X, Deng L, Wang D, Li N, Chen X, Cheng X, et al. Mechanism of TNF-α autocrine effects in hypoxic cardiomyocytes: Initiated by hypoxia inducible factor 1α, presented by exosomes. J Mol Cell Cardiol. 2012;53(6):848-57.
https://doi.org/10.1016/j.yjmcc.2012.10.002
Seclì L, Sorge M, Morotti A, Brancaccio M. Blocking Extracellular Chaperones to Improve Cardiac Regeneration. Front Bioeng Biotechnol. 2020;8:411.
https://doi.org/10.3389/fbioe.2020.00411
Zhang H, Liu J, Qu D, Wang L, Wong CM, Lau C-W, et al. Serum exosomes mediate delivery of arginase 1 as a novel mechanism for endothelial dysfunction in diabetes. Proc Natl Acad Sci U S A. 2018;115(29):E6927-E36.
https://doi.org/10.1073/pnas.1721521115
Del Re DP, Matsuda T, Zhai P, Maejima Y, Jain MR, Liu T, et al. Mst1 promotes cardiac myocyte apoptosis through phosphorylation and inhibition of Bcl-xL. Mol cell. 2014;54(4):639-50.
https://doi.org/10.1016/j.molcel.2014.04.007
Odashima M, Usui S, Takagi H, Hong C, Liu J, Yokota M, et al. Inhibition of Endogenous Mst1 Prevents Apoptosis and Cardiac Dysfunction Without Affecting Cardiac Hypertrophy After Myocardial Infarction. Circ res. 2007;100(9):1344-52.
https://doi.org/10.1161/01.RES.0000265846.23485.7a
Borén J, Packard CJ, Taskinen M-R. The Roles of ApoC-III on the Metabolism of Triglyceride-Rich Lipoproteins in Humans. Front Endocrinol. 2020;11:474.
https://doi.org/10.3389/fendo.2020.00474
Oestvang J, Bonnefont-Rousselot D, Ninio E, Hakala JK, Johansen B, Anthonsen MW. Modification of LDL with human secretory phospholipase A(2) or sphingomyelinase promotes its arachidonic acid-releasing propensity. J Lipid Res. 2004;45(5):831-8.
https://doi.org/10.1194/jlr.M300310-JLR200
Rizzo M, Carlo-Stella C. Arachidonic acid mediates interleukin-1 and tumor necrosis factor-alpha- induced activation of the c-jun amino-terminal kinases in stromal cells. Blood. 1996;88(10):3792-800.
https://doi.org/10.1182/blood.V88.10.3792.bloodjournal88103792
Kawakami A, Aikawa M, Alcaide P, Luscinskas FW, Libby P, Sacks FM. Apolipoprotein CIII induces expression of vascular cell adhesion molecule-1 in vascular endothelial cells and increases adhesion of monocytic cells. Circulation. 2006;114(7):681-7.
https://doi.org/10.1161/CIRCULATIONAHA.106.622514
Gong T, Zhou R. ApoC3: an 'alarmin' triggering sterile inflammation. Nat Immunol. 2020;21(1):9-11.
https://doi.org/10.1038/s41590-019-0562-3
Ailawadi S, Wang X, Gu H, Fan G-C. Pathologic function and therapeutic potential of exosomes in cardiovascular disease. Biochim Biophys Acta. 2015;1852(1):1-11.
https://doi.org/10.1016/j.bbadis.2014.10.008
Li M, Wang J, Guo P, Jin L, Tan X, Zhang Z, et al. Exosome mimetics derived from bone marrow mesenchymal stem cells ablate neuroblastoma tumor in vitro and in vivo. Biomater Adv. 2022;142:213161.
https://doi.org/10.1016/j.bioadv.2022.213161
Nie X, Fan J, Li H, Yin Z, Zhao Y, Dai B, et al. miR-217 Promotes Cardiac Hypertrophy and Dysfunction by Targeting PTEN. Mol Ther Nucleic Acids. 2018;12:254-66.
https://doi.org/10.1016/j.omtn.2018.05.013
Wang X, Huang W, Liu G, Cai W, Millard RW, Wang Y, et al. Cardiomyocytes mediate anti-angiogenesis in type 2 diabetic rats through the exosomal transfer of miR-320 into endothelial cells. J Mol Cell Cardiol. 2014;74:139-50.
https://doi.org/10.1016/j.yjmcc.2014.05.001
Shen W, Lu Y, Hu J, Le H, Yu W, Xu W, et al. Mechanism of miR-320 in Regulating Biological Characteristics of Ischemic Cerebral Neuron by Mediating Nox2/ROS Pathway. J Mol Neurosci : MN. 2020;70(3):449-57.
https://doi.org/10.1007/s12031-019-01434-5
Halkein J, Tabruyn SP, Ricke-Hoch M, Haghikia A, Nguyen N-Q-N, Scherr M, et al. MicroRNA-146a is a therapeutic target and biomarker for peripartum cardiomyopathy. J Clin Invest. 2013;123(5):2143-54.
https://doi.org/10.1172/JCI64365
Su Q, Xu Y, Cai R, Dai R, Yang X, Liu Y, et al. miR-146a inhibits mitochondrial dysfunction and myocardial infarction by targeting cyclophilin D. Mol Ther Nucleic Acids. 2021;23:1258-71.
https://doi.org/10.1016/j.omtn.2021.01.034
Xue S, Zhu W, Liu D, Su Z, Zhang L, Chang Q, et al. Circulating miR-26a-1, miR-146a and miR-199a-1 are potential candidate biomarkers for acute myocardial infarction. Mol Med. 2019;25(1):18.
https://doi.org/10.1186/s10020-019-0086-1
Fang X, Stroud MJ, Ouyang K, Fang L, Zhang J, Dalton ND, et al. Adipocyte-specific loss of PPARγ attenuates cardiac hypertrophy. JCI insight. 2016;1(16):e89908.
https://doi.org/10.1172/jci.insight.89908
Yan H, Wang H, Zhu X, Huang J, Li Y, Zhou K, et al. Adeno-associated virus-mediated delivery of anti-miR-199a tough decoys attenuates cardiac hypertrophy by targeting PGC-1alpha. Mol Ther Nucleic Acids. 2021;23:406-17.
https://doi.org/10.1016/j.omtn.2020.11.007
Chen D, Li Z, Bao P, Chen M, Zhang M, Yan F, et al. Nrf2 deficiency aggravates Angiotensin II-induced cardiac injury by increasing hypertrophy and enhancing IL-6/STAT3-dependent inflammation. Biochim Biophys Acta Mol Basis Dis. 2019;1865(6):1253-64.
https://doi.org/10.1016/j.bbadis.2019.01.020
Liu ZH, Zhang Y, Wang X, Fan XF, Zhang Y, Li X, et al. SIRT1 activation attenuates cardiac fibrosis by endothelial-to-mesenchymal transition. Biomed Pharmacother. 2019;118:109227.
https://doi.org/10.1016/j.biopha.2019.109227
Ponnusamy M, Li P-F, Wang K. Understanding cardiomyocyte proliferation: an insight into cell cycle activity. Cell Mol Life Sci. 2017;74:1019-34.
https://doi.org/10.1007/s00018-016-2375-y
Zeng N, Huang Y-Q, Yan Y-M, Hu Z-Q, Zhang Z, Feng J-X, et al. Diverging targets mediate the pathological roleof miR-199a-5p and miR-199a-3p by promoting cardiac hypertrophy and fibrosis. Mol Ther Nucleic Acids. 2021;26:1035-50.
https://doi.org/10.1016/j.omtn.2021.10.013
Xue R, Tan W, Wu Y, Dong B, Xie Z, Huang P, et al. Role of Exosomal miRNAs in Heart Failure. Front Cardiovasc Med. 2020;7(347):592412.
https://doi.org/10.3389/fcvm.2020.592412
Hinrichsen R, Haunsø S, Busk PK. Different regulation of p27 and Akt during cardiomyocyte proliferation and hypertrophy. Growth factors (Chur, Switzerland). 2007;25(2):132-40.
https://doi.org/10.1080/08977190701549835
Eom G, Cho Y, Ko J-H, Shin S, Choe N, Kim Y, et al. Casein Kinase-2 1 Induces Hypertrophic Response by Phosphorylation of Histone Deacetylase 2 S394 and its Activation in the Heart. Circulation. 2011;123:2392-403.
https://doi.org/10.1161/CIRCULATIONAHA.110.003665
Su M, Wang J, Wang C, Wang X, Dong W, Qiu W, et al. Correction: MicroRNA-221 inhibits autophagy and promotes heart failure by modulating the p27/CDK2/mTOR axis. Cell Death Differ. 2021;28(1):420-2.
https://doi.org/10.1038/s41418-020-0582-4
Hullinger TG, Montgomery RL, Seto AG, Dickinson BA, Semus HM, Lynch JM, et al. Inhibition of miR-15 Protects Against Cardiac Ischemic Injury. Circ res. 2012;110(1):71-81.
https://doi.org/10.1161/CIRCRESAHA.111.244442
Zhu H, Yang Y, Wang Y, Li J, Schiller PW, Peng T. MicroRNA-195 promotes palmitate-induced apoptosis in cardiomyocytes by down-regulating Sirt1. Cardiovasc Res. 2011;92(1):75-84.
https://doi.org/10.1093/cvr/cvr145
Ni YG, Berenji K, Wang N, Oh M, Sachan N, Dey A, et al. Foxo Transcription Factors Blunt Cardiac Hypertrophy by Inhibiting Calcineurin Signaling. Circulation. 2006;114(11):1159-68.
https://doi.org/10.1161/CIRCULATIONAHA.106.637124
Yuan XN, Zhang J, Xie FF, Tan WQ, Wang ST, Huang L, et al. Loss of the Protein Cystathionine beta-Synthase During Kidney Injury Promotes Renal Tubulointerstitial Fibrosis. Kidney Blood Press Res. 2017;42(3):428-43.
https://doi.org/10.1159/000479295
Xu K, Chen C, Wu Y, Wu M, Lin L. Advances in miR-132-Based Biomarker and Therapeutic Potential in the Cardiovascular System. Front Pharmacol. 2021;12:751487.
https://doi.org/10.3389/fphar.2021.751487
Ding J, Tang Q, Luo B, Zhang L, Lin L, Han L, et al. Klotho inhibits angiotensin II-induced cardiac hypertrophy, fibrosis, and dysfunction in mice through suppression of transforming growth factor-β1 signaling pathway. Eur J Pharmacol. 2019;859:172549.
https://doi.org/10.1016/j.ejphar.2019.172549
Sengupta A, Molkentin JD, Yutzey KE. FoxO transcription factors promote autophagy in cardiomyocytes. J Biol Chem. 2009;284(41):28319-31.
https://doi.org/10.1074/jbc.M109.024406
Abdellatif M, Sedej S, Carmona-Gutierrez D, Madeo F, Kroemer G. Autophagy in Cardiovascular Aging. Circ res. 2018;123(7):803-24.
https://doi.org/10.1161/CIRCRESAHA.118.312208
Ucar A, Gupta SK, Fiedler J, Erikci E, Kardasinski M, Batkai S, et al. The miRNA-212/132 family regulates both cardiac hypertrophy and cardiomyocyte autophagy. Nat Commun. 2012;3(1):1078.
https://doi.org/10.1038/ncomms2090
Yabluchanskiy A, Ma Y, Iyer RP, Hall ME, Lindsey ML. Matrix metalloproteinase-9: Many shades of function in cardiovascular disease. Physiology (Bethesda). 2013;28(6):391-403.
https://doi.org/10.1152/physiol.00029.2013
Jiang X, Ning Q, Wang J. Angiotensin II induced differentially expressed microRNAs in adult rat cardiac fibroblasts. J Physiol Sci. 2013;63(1):31-8.
https://doi.org/10.1007/s12576-012-0230-y
Schimmel K, Stojanović SD, Huang CK, Jung M, Meyer MH, Xiao K, et al. Combined high-throughput library screening and next generation RNA sequencing uncover microRNAs controlling human cardiac fibroblast biology. J Mol Cell Cardiol. 2021;150:91-100.
https://doi.org/10.1016/j.yjmcc.2020.10.008
Lei Z, Wahlquist C, El Azzouzi H, Deddens JC, Kuster D, van Mil A, et al. miR-132/212 Impairs Cardiomyocytes Contractility in the Failing Heart by Suppressing SERCA2a. Front Cardiovasc Med. 2021;8:592362-.
https://doi.org/10.3389/fcvm.2021.592362
Haybar H, Shahrabi S, Rezaeeyan H, Shirzad R, Saki N. Protective role of heat shock transcription factor 1 in heart failure: A diagnostic approach. J Cell Physiol. 2019 Jun;234(6):7764-7770.
https://doi.org/10.1002/jcp.27639
Crackower MA, Oudit GY, Kozieradzki I, Sarao R, Sun H, Sasaki T, et al. Regulation of myocardial contractility and cell size by distinct PI3K-PTEN signaling pathways. Cell. 2002;110(6):737-49.
https://doi.org/10.1016/S0092-8674(02)00969-8
Rai A, Whaley-Connell A, McFarlane S, Sowers JR. Hyponatremia, Arginine Vasopressin Dysregulation, and Vasopressin Receptor Antagonism. Am J Nephrol. 2006;26(6):579-89.
https://doi.org/10.1159/000098028
Bijkerk R, Trimpert C, Solingen Cv, Bruin RGd, Florijn BW, Kooijman S, et al. MicroRNA-132 controls water homeostasis through regulating MECP2-mediated vasopressin synthesis. Am J Physiol Renal Physiol. 2018;315(4):F1129-F38.
https://doi.org/10.1152/ajprenal.00087.2018
Eshraghi N, Jamal A, Eshraghi N, Kashanian M, Sheikhansari N. Cerebroplacental ratio (CPR) and reduced fetal movement: predicting neonatal outcomes. J Matern Fetal Neonatal Med. 2022 May;35(10):1923-1928.
https://doi.org/10.1080/14767058.2020.1774544
Hinkel R, Batkai S, Bähr A, Bozoglu T, Straub S, Borchert T, et al. AntimiR-132 Attenuates Myocardial Hypertrophy in an Animal Model of Percutaneous Aortic Constriction. J Am Coll Cardiol. 2021;77(23):2923-35.
https://doi.org/10.1016/j.jacc.2021.04.028
Zhou Y, Li KS, Liu L, Li SL. MicroRNA‑132 promotes oxidative stress‑induced pyroptosis by targeting sirtuin 1 in myocardial ischaemia‑reperfusion injury. Int J Mol Med. 2020;45(6):1942-50.
https://doi.org/10.3892/ijmm.2020.4557
Li H, Zhang P, Li F, Yuan G, Wang X, Zhang A, et al. Plasma miR-22-5p, miR-132-5p, and miR-150-3p Are Associated with Acute Myocardial Infarction. Biomed Res Int. 2019;2019:5012648.
https://doi.org/10.1155/2019/5012648
Azarmehr N, Porhemat R, Roustaei N, Radmanesh E, Moslemi Z, Vanda R, Barmoudeh Z, Eslamnik P, Doustimotlagh AH. Melatonin-Attenuated Oxidative Stress in High-Risk Pregnant Women Receiving Enoxaparin and Aspirin. Evid Based Complement Alternat Med. 2023 May 25;2023:9523923.
https://doi.org/10.1155/2023/9523923
E L, Jiang H, Lu Z. MicroRNA-144 attenuates cardiac ischemia/reperfusion injury by targeting FOXO1. Exp Ther Med. 2019;17(3):2152-60.
https://doi.org/10.3892/etm.2019.7161
Milano G, Biemmi V, Lazzarini E, Balbi C, Ciullo A, Bolis S, et al. Intravenous administration of cardiac progenitor cell-derived exosomes protects against doxorubicin/trastuzumab-induced cardiac toxicity. Cardiovasc Res. 2019;116(2):383-92.
https://doi.org/10.1093/cvr/cvz108
Mayourian J, Ceholski DK, Gorski PA, Mathiyalagan P, Murphy JF, Salazar SI, et al. Exosomal microRNA-21-5p Mediates Mesenchymal Stem Cell Paracrine Effects on Human Cardiac Tissue Contractility. Circ res. 2018;122(7):933-44.
https://doi.org/10.1161/CIRCRESAHA.118.312420
Barile L, Lionetti V, Cervio E, Matteucci M, Gherghiceanu M, Popescu LM, et al. Extracellular vesicles from human cardiac progenitor cells inhibit cardiomyocyte apoptosis and improve cardiac function after myocardial infarction. Cardiovasc Res. 2014;103(4):530-41.
https://doi.org/10.1093/cvr/cvu167
Li Q, Xie J, Li R, Shi J, Sun J, Gu R, et al. Overexpression of microRNA-99a attenuates heart remodelling and improves cardiac performance after myocardial infarction. J Cell Mol Med. 2014;18(5):919-28.
https://doi.org/10.1111/jcmm.12242
Couto Gd, Gallet R, Cambier L, Jaghatspanyan E, Makkar N, Dawkins JF, et al. Exosomal MicroRNA Transfer Into Macrophages Mediates Cellular Postconditioning. Circulation. 2017;136(2):200-14.
https://doi.org/10.1161/CIRCULATIONAHA.116.024590
Arslan F, Lai RC, Smeets MB, Akeroyd L, Choo A, Aguor ENE, et al. Mesenchymal stem cell-derived exosomes increase ATP levels, decrease oxidative stress and activate PI3K/Akt pathway to enhance myocardial viability and prevent adverse remodeling after myocardial ischemia/reperfusion injury. Stem Cell Res. 2013;10(3):301-12.
https://doi.org/10.1016/j.scr.2013.01.002
Qian R, Jing B, Jiang D, Gai Y, Zhu Z, Huang X, et al. Multi-antitumor therapy and synchronous imaging monitoring based on exosome. Eur J Nucl Med Mol Imaging. 2022;49(8):2668-81.
https://doi.org/10.1007/s00259-022-05696-x
Yu B, Kim HW, Gong M, Wang J, Millard RW, Wang Y, et al. Exosomes secreted from GATA-4 overexpressing mesenchymal stem cells serve as a reservoir of anti-apoptotic microRNAs for cardioprotection. Int J Cardiol. 2015;182:349-60.
https://doi.org/10.1016/j.ijcard.2014.12.043
Ansari MA, Thiruvengadam M, Venkidasamy B, Alomary MN, Salawi A, Chung I-M, et al. Exosome-based nanomedicine for cancer treatment by targeting inflammatory pathways: current status and future perspectives. Semin Cancer Biol. 2022: Elsevier.
https://doi.org/10.1016/j.semcancer.2022.04.005
Gao F, Kataoka M, Liu N, Liang T, Huang Z-P, Gu F, et al. Therapeutic role of miR-19a/19b in cardiac regeneration and protection from myocardial infarction. Nat Commun. 2019;10(1):1802.
https://doi.org/10.1038/s41467-019-09530-1
Lee DI, Kass DA. Phosphodiesterases and cyclic GMP regulation in heart muscle. Physiology. 2012;27 4:248-58.
https://doi.org/10.1152/physiol.00011.2012
Liu K, Hao Q, Wei J, Li G-H, Wu Y, Zhao Y-F. MicroRNA-19a/b-3p protect the heart from hypertension-induced pathological cardiac hypertrophy through PDE5A. J Hypertens. 2018;36(9):1847-57.
https://doi.org/10.1097/HJH.0000000000001769
Zhang M, Koitabashi N, Nagayama T, Rambaran R, Feng N, Takimoto E, et al. Expression, activity, and pro-hypertrophic effects of PDE5A in cardiac myocytes. Cell Signal. 2008;20(12):2231-6.
https://doi.org/10.1016/j.cellsig.2008.08.012
Feng Y, Huang W, Wani M, Yu X, Ashraf M. Ischemic preconditioning potentiates the protective effect of stem cells through secretion of exosomes by targeting Mecp2 via miR-22. PLoS One. 2014;9(2):e88685-e.
https://doi.org/10.1371/journal.pone.0088685
Yu B, Gong M, Wang Y, Millard RW, Pasha Z, Yang Y, et al. Cardiomyocyte protection by GATA-4 gene engineered mesenchymal stem cells is partially mediated by translocation of miR-221 in microvesicles. PLoS One. 2013;8(8):e73304.
https://doi.org/10.1371/journal.pone.0073304
Venkat P, Cui C, Chen Z, Chopp M, Zacharek A, Landschoot-Ward J, et al. CD133+Exosome Treatment Improves Cardiac Function after Stroke in Type 2 Diabetic Mice. Transl Stroke Res. 2021;12(1):112-24.
https://doi.org/10.1007/s12975-020-00807-y
Fish J, Santoro M, Morton S, Yu S, Yeh R-F, Wythe J, et al. miR-126 Regulates Angiogenic Signaling and Vascular Integrity. Dev Cell. 2008;15:272-84.
https://doi.org/10.1016/j.devcel.2008.07.008
Mondadori dos Santos A, Metzinger L, Haddad O, M'Baya-Moutoula E, Taïbi F, Charnaux N, et al. miR-126 Is Involved in Vascular Remodeling under Laminar Shear Stress. Biomed Res Int. 2015;2015:497280.
https://doi.org/10.1155/2015/497280
Wang Y, Wang F, Wu Y, Zuo L, Zhang S, Zhou Q, et al. MicroRNA-126 attenuates palmitate-induced apoptosis by targeting TRAF7 in HUVECs. Mol Cell Biochem. 2015;399(1):123-30.
https://doi.org/10.1007/s11010-014-2239-4
https://doi.org/10.1007/s11010-015-2460-9
Chistiakov DA, Orekhov AN, Bobryshev YV. The role of miR-126 in embryonic angiogenesis, adult vascular homeostasis, and vascular repair and its alterations in atherosclerotic disease. J Mol Cell Cardiol. 2016;97:47-55.
https://doi.org/10.1016/j.yjmcc.2016.05.007
Wang X, Gu H, Qin D, Yang L, Huang W, Essandoh K, et al. Exosomal miR-223 Contributes to Mesenchymal Stem Cell-Elicited Cardioprotection in Polymicrobial Sepsis. Sci Rep. 2015;5(1):13721.
https://doi.org/10.1038/srep13721
Qin D, Wang X, Li Y, Yang L, Wang R, Peng J, et al. MicroRNA-223-5p and -3p Cooperatively Suppress Necroptosis in Ischemic/Reperfused Hearts * . J Biol Chem. 2016;291(38):20247-59.
https://doi.org/10.1074/jbc.M116.732735
Zhang M-W, Shen Y-J, Shi J, Yu J-G. MiR-223-3p in Cardiovascular Diseases: A Biomarker and Potential Therapeutic Target. Front Cardiovasc Med. 2021;7:610561-.
https://doi.org/10.3389/fcvm.2020.610561
Fasanaro P, Greco S, Lorenzi M, Pescatori M, Brioschi M, Kulshreshtha R, et al. An Integrated Approach for Experimental Target Identification of Hypoxia-induced miR-210. J Biol Chem. 2009;284:35134-43.
https://doi.org/10.1074/jbc.M109.052779
Hakemi MS, Nassiri AA, Nobakht A, Mardani M, Darazam IA, Parsa M, Miri MM, Shahrami R, Koomleh AA, Entezarmahdi K, Karimi A. Benefit of Hemoadsorption Therapy in Patients Suffering Sepsis-Associated Acute Kidney Injury: A Case Series. Blood Purif. 2022;51(10):823-830.
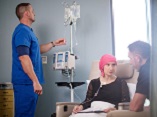
Published
Issue
Section
License
Copyright (c) 2023 Galen Medical Journal

This work is licensed under a Creative Commons Attribution 4.0 International License.