The Role of Epigenetics in Myocardial Infarction: Mechanism and Therapeutic Potential
The Role of Epigenetics in Myocardial Infarction
DOI:
https://doi.org/10.31661/gmj.v13i.3474Keywords:
Myocardial Infarction; Epigenetics; DNA Methylation; Noncoding RNAs; Cardiac Repair.Abstract
Myocardial infarction (MI), remains one of the leading causes of morbidity and mortality worldwide. As a result, understanding the underlying mechanisms of MI is crucial for developing effective therapeutic strategies. Epigenetics, which involves heritable changes in gene expression without altering the underlying DNA sequence, has emerged as a significant factor in the pathogenesis and progression of MI. Key epigenetic mechanisms such as DNA methylation, histone modifications, and noncoding RNAs (ncRNAs) have been shown to regulate genes associated with inflammation, apoptosis, fibrosis, and cardiac repair. These epigenetic alterations contribute to the complex gene-environment interactions that influence clinical outcomes in MI patients. Recent research has identified specific epigenetic changes that can serve as biomarkers for MI risk stratification, offering potential for early diagnosis and personalized therapeutic interventions. Moreover, targeting these epigenetic modifications holds promise as a therapeutic strategy to reduce myocardial damage, enhance cardiac function, and prevent adverse remodeling after MI. This review explores the mechanisms by which epigenetic regulation influences MI pathogenesis and discusses the therapeutic potential of targeting these pathways to improve patient outcomes. By integrating epigenetic therapies into clinical practice, it may be possible to revolutionize the treatment of MI, addressing the disease at its molecular roots and offering more effective, durable interventions.
References
Jayaraj J, Davatyan K, Subramanian S, Priya J. Epidemiology of Myocardial Infarction. Myocardial Infarction. 2018;10:9-17.
Saleh M, Ambrose JA. Understanding myocardial infarction. F1000Res. 2018 Sep 3;7:F1000 Faculty Rev-1378.
https://doi.org/10.12688/f1000research.15096.1
PMid:30228871 PMCid:PMC6124376
Cardiovascular diseases. (CVDs). cited 2024 : Aug 30; Available from: https://www.who.int/news-room/fact-sheets/detail/cardiovascular-diseases-(cvds)
GBD 2017 Causes of Death Collaborators. Global, regional, and national age-sex-specific mortality for 282 causes of death in 195 countries and territories, 1980-2017: a systematic analysis for the Global Burden of Disease Study 2017. Lancet. 2018 Nov 10;392(10159):1736-88.
Kaptoge S, Pennells L, Bacquer DD, Cooney MT, Kavousi M, Stevens G, et al. World Health Organization cardiovascular disease risk charts: revised models to estimate risk in 21 global regions.[preprint]. The Lancet Global Health. 2019 Oct 1;7(10):e1332-45.
Schleithoff C, Voelter-Mahlknecht S, Dahmke IN, Mahlknecht U. On the epigenetics of vascular regulation and disease. Clin Epigenet. 2012 Dec;4(1):7.
https://doi.org/10.1186/1868-7083-4-7
PMid:22621747 PMCid:PMC3438017
Di Salvo TD, Haldar SM. Epigenetic Mechanisms in Heart Failure Pathogenesis. Circulation: Heart Failure. 2014;7:850-63.
https://doi.org/10.1161/CIRCHEARTFAILURE.114.001193
PMid:25228320 PMCid:PMC4169025
Choi S, Jung Y. Epigenetic Mechanisms of Cardiovascular Aging. Journal of cardiovascular translational research. 2015;8:248-58.
Xie L, Zhang Q, Mao J, Zhang J, Li L. The Roles of lncRNA in Myocardial Infarction: Molecular Mechanisms, Diagnosis Biomarkers, and Therapeutic Perspectives. Frontiers in Cell and Developmental Biology. 2021;9:680713.
https://doi.org/10.3389/fcell.2021.680713
PMid:34604208 PMCid:PMC8481623
Xu R, Zhang L, Song Q, Ji H, Wu S, Li X, et al. miRNA-Mediated Epigenetic Regulation in Cardiovascular Disease. Journal of Molecular and Cellular Cardiology. 2019;130:121-34.
Salvatori F, D'Aversa E, Serino M, Singh AV, Secchiero P, Zauli G, et al. miRNAs Epigenetic Tuning of Wall Remodeling in the Early Phase after Myocardial Infarction. A Novel Epidrug Approach: International Journal of Molecular Sciences; Available from: https://consensus.app/papers/mirnas-epigenetic-tuning-wall-remodeling-early-phase-salvatori/10efd8d43cf15f8f8f3f1569819787b9/?utm_source=chatgpt
Han Y, Duan B, Wu J, Zheng Y, Gu Y, Cai X, et al. Analysis of Time Series Gene Expression and DNA Methylation Reveals the Molecular Features of Myocardial Infarction Progression. Front Cardiovasc Med. 2022;9:912454.
https://doi.org/10.3389/fcvm.2022.912454
PMid:35811717 PMCid:PMC9263976
Turunen M, Husso T, Musthafa HSN, Laidinen S, Dragneva G, Laham-Karam N, et al. Epigenetic Upregulation of Endogenous VEGF-A Reduces Myocardial Infarct Size in Mice. PLoS ONE. 2014 ;9(2):e89979.
https://doi.org/10.1371/journal.pone.0089979
PMid:24587164 PMCid:PMC3935957
Kim M, Yin P, Tu T, Xu C, Kim D, Yang Y, et al. Epigenetic Mechanisms and Their Implications in Cardiovascular Disease. Circulation Research. 2018;123:137-53.
Chikh G, Jong SD de, Sekirov L, Raney S, Kazem M, Wilson KD, et al. Synthetic methylated CpG ODNs are potent in vivo adjuvants when delivered in liposomal nanoparticles. International immunology. 2009;21 7:757-67.
https://doi.org/10.1093/intimm/dxp044
PMid:19502586
Smith ZD, Meissner A. DNA methylation: roles in mammalian development. Nat Rev Genet. 2013 Mar;14(3):204-20.
https://doi.org/10.1038/nrg3354
PMid:23400093
Luger A, Zhou C, Kyriakakis P, So J, David C, Heier J, et al. DNA Methylation and Cardiovascular Disease. Trends in Cardiovascular Medicine. 2015;25:68-77.
Pepin ME, Drakos S, Ha CM, Tristani-Firouzi M, Selzman CH, Fang JC, et al. DNA methylation reprograms cardiac metabolic gene expression in end-stage human heart failure. American Journal of Physiology-Heart and Circulatory Physiology. 2019 Oct 1;317(4):H674-84.
https://doi.org/10.1152/ajpheart.00016.2019
PMid:31298559 PMCid:PMC6843013
Talens RP, Jukema JW, Trompet S, Kremer D, Westendorp RGJ, Lumey LH, et al. Hypermethylation at loci sensitive to the prenatal environment is associated with increased incidence of myocardial infarction. Int J Epidemiol. 2012 Feb;41(1):106-15.
https://doi.org/10.1093/ije/dyr153
PMid:22101166 PMCid:PMC3663184
Luttmer R, Spijkerman A, Kok R, Jakobs C, Blom H, Serné E, et al. Metabolic syndrome components are associated with DNA hypomethylation. Obesity research & clinical practice. 2013;7 2:106-15.
https://doi.org/10.1016/j.orcp.2012.06.001
PMid:24331772
Smith J, Sen S, Weeks R, Eccles M, Chatterjee A. Promoter DNA Hypermethylation and Paradoxical Gene Activation. Trends in cancer. 2020;6 5:392-406.
https://doi.org/10.1016/j.trecan.2020.02.007
PMid:32348735
Chen H, Orozco LD, Wang J, Rau CD, Rubbi L, Ren S, et al. DNA Methylation Indicates Susceptibility to Isoproterenol-Induced Cardiac Pathology and Is Associated With Chromatin States. Circ Res. 2016 Mar 4;118(5):786-97.
https://doi.org/10.1161/CIRCRESAHA.115.305298
PMid:26838786 PMCid:PMC4779427
Krishna SM, Golledge J. The role of thrombospondin-1 in cardiovascular health and pathology. International Journal of Cardiology. 2013 Sep 30;168(2):692-706.
https://doi.org/10.1016/j.ijcard.2013.04.139
PMid:23664438
Thal MA, Krishnamurthy P, Mackie AR, Hoxha E, Lambers E, Verma S, et al. Enhanced Angiogenic and Cardiomyocyte Differentiation Capacity of Epigenetically Reprogrammed Mouse and Human Endothelial Progenitor Cells Augments Their Efficacy for Ischemic Myocardial Repair. Circulation Research. 2012 Jul 6;111(2):180-90.
https://doi.org/10.1161/CIRCRESAHA.112.270462
PMid:22589372 PMCid:PMC3406600
Tarazón E, Pérez-Carrillo L, Giménez-Escamilla I, García-Manzanares M, Martínez-Dolz L, Portolés M, et al. DNMT3B System Dysregulation Contributes to the Hypomethylated State in Ischaemic Human Hearts. Biomedicines. 2022 Apr;10(4):866.
https://doi.org/10.3390/biomedicines10040866
PMid:35453616 PMCid:PMC9029641
Lee JS, Chang PY, Zhang Y, Kizer J, Best L, Howard B. Triglyceride and HDL-C Dyslipidemia and Risks of Coronary Heart Disease and Ischemic Stroke by Glycemic Dysregulation Status: The Strong Heart Study. Diabetes Care. 2017;40:529-37.
https://doi.org/10.2337/dc16-1958
PMid:28122840 PMCid:PMC5360283
Chen J, Liu Z, Ma L, Gao S, Fu H, Wang C, et al. Targeting Epigenetics and Non-coding RNAs in Myocardial Infarction: From Mechanisms to Therapeutics. Frontiers in Genetics. 2021;12:780649.
https://doi.org/10.3389/fgene.2021.780649
PMid:34987550 PMCid:PMC8721121
Wang K, Li Y, Qiang T, Chen J, Wang X. Role of epigenetic regulation in myocardial ischemia/reperfusion injury. Pharmacological Research. 2021 Aug;170:105743.
https://doi.org/10.1016/j.phrs.2021.105743
PMid:34182132
Turner BM. Histone acetylation and an epigenetic code. Bioessays. 2000 Aug 10;22(9):836-45.
https://doi.org/10.1002/1521-1878(200009)22:9<836::AID-BIES9>3.0.CO;2-X
PMid:10944586
Struhl K. Histone acetylation and transcriptional regulatory mechanisms. Genes & Development. 1998 Mar 1;12(5):599-606.
https://doi.org/10.1101/gad.12.5.599
PMid:9499396
Wang Y, Miao X, Liu Y, Li F, Liu Q, Sun J, et al. Dysregulation of histone acetyltransferases and deacetylases in cardiovascular diseases. Oxid Med Cell Longev. 2014;2014:641979.
https://doi.org/10.1155/2014/641979
PMid:24693336 PMCid:PMC3945289
Funamoto M, Sunagawa Y, Katanasaka Y, Shimizu K, Miyazaki Y, Sari N, et al. Histone Acetylation Domains Are Differentially Induced during Development of Heart Failure in Dahl Salt-Sensitive Rats. International Journal of Molecular Sciences. 2021 Jan;22(4):1771.
https://doi.org/10.3390/ijms22041771
PMid:33578969 PMCid:PMC7916721
Ferreira JP, Pitt B, Zannad F. Histone deacetylase inhibitors for cardiovascular conditions and healthy longevity. The Lancet Healthy Longevity. 2021 Jun 1;2(6):e371-9.
https://doi.org/10.1016/S2666-7568(21)00061-1
PMid:36098145
Zhang LX, Qin X, Zhao Y, Fast L, Zhuang S, Liu PY, et al. Inhibition of Histone Deacetylases Preserves Myocardial Performance and Prevents Cardiac Remodeling through Stimulation of Endogenous Angiomyogenesis. Journal of Pharmacology and Experimental Therapeutics. 2012;341:285-93.
https://doi.org/10.1124/jpet.111.189910
PMid:22271820 PMCid:PMC3310703
Kong Y, Tannous P, Lu G, Berenji K, Rothermel BA, Olson EN, et al. Suppression of class I and II histone deacetylases blunts pressure-overload cardiac hypertrophy. Circulation. 2006 Jun 6;113(22):2579-88.
https://doi.org/10.1161/CIRCULATIONAHA.106.625467
PMid:16735673 PMCid:PMC4105979
Karamboulas C, Swedani A, Ward C, Al-Madhoun AS, Wilton S, Boisvenue S, et al. HDAC activity regulates entry of mesoderm cells into the cardiac muscle lineage. Journal of Cell Science. 2006 Oct 15;119(20):4305-14.
https://doi.org/10.1242/jcs.03185
PMid:17038545
Renaud L, Harris LG, Mani SK, Kasiganesan H, Chou JC, Baicu CF, et al. HDACs Regulate miR-133a Expression in Pressure Overload-Induced Cardiac Fibrosis. Circ: Heart Failure. 2015 Nov;8(6):1094-104.
https://doi.org/10.1161/CIRCHEARTFAILURE.114.001781
PMid:26371176 PMCid:PMC4651803
Yi X, Zhu QX, Wu XL, Tan TT, Jiang XJ. Histone Methylation and Oxidative Stress in Cardiovascular Diseases. Oxidative Medicine and Cellular Longevity. 2022;2022(1):6023710.
https://doi.org/10.1155/2022/6023710
PMid:35340204 PMCid:PMC8942669
Wamstad JA, Alexander JM, Truty RM, Shrikumar A, Li F, Eilertson KE, et al. Dynamic and Coordinated Epigenetic Regulation of Developmental Transitions in the Cardiac Lineage. Cell. 2012 Sep 28;151(1):206-20.
https://doi.org/10.1016/j.cell.2012.07.035
PMid:22981692 PMCid:PMC3462286
Zhu J yi, van de Leemput J, Han Z. The Roles of Histone Lysine Methyltransferases in Heart Development and Disease. Journal of Cardiovascular Development and Disease. 2023 Jul;10(7):305.
https://doi.org/10.3390/jcdd10070305
PMid:37504561 PMCid:PMC10380575
Ni L, Lin B, Zhang Y, Hu L, Lin J, Fu F, et al. Histone modification landscape and the key significance of H3K27me3 in myocardial ischaemia/reperfusion injury. Sci China Life Sci. 2023 Jun 1;66(6):1264-79.
https://doi.org/10.1007/s11427-022-2257-9
PMid:36808292
Lemon DD, Horn TR, Cavasin MA, Jeong MY, Haubold KW, Long CS, et al. Cardiac HDAC6 Catalytic Activity is Induced in Response to Chronic Hypertension. J Mol Cell Cardiol. 2011 Jul;51(1):41-50.
https://doi.org/10.1016/j.yjmcc.2011.04.005
PMid:21539845 PMCid:PMC3113526
Fadaei S, Zarepour F, Parvaresh M, Motamedzadeh A, Zadeh SST, Sheida A, et al. Epigenetic regulation in myocardial infarction: Non-coding RNAs and exosomal non-coding RNAs. Frontiers in Cardiovascular Medicine. 2022;9:1014961.
https://doi.org/10.3389/fcvm.2022.1014961
PMid:36440025 PMCid:PMC9685618
Pérez-Cremades D. MicroRNA-mediated control of myocardial infarction in diabetes. Trends in Cardiovascular Medicine. 2023 May 1;33(4):195-201.
https://doi.org/10.1016/j.tcm.2022.01.004
PMid:35051592 PMCid:PMC9288556
Cheng C, Wang Q, You W, Chen M, Xia J. MiRNAs as Biomarkers of Myocardial Infarction: A Meta-Analysis. PLoS ONE. 2014;9(2):e88566.
https://doi.org/10.1371/journal.pone.0088566
PMid:24533109 PMCid:PMC3922900
Takaya T, Ono K, Kawamura T, Takanabe R, Kaichi S, Morimoto T, et al. MicroRNA-1 and MicroRNA-133 in spontaneous myocardial differentiation of mouse embryonic stem cells. Circ J. 2009 Aug;73(8):1492-7.
https://doi.org/10.1253/circj.CJ-08-1032
PMid:19521018
Izarra A, Moscoso I, Cañón S, Carreiro C, Fondevila D, Martín-Caballero J, et al. miRNA-1 and miRNA-133a are involved in early commitment of pluripotent stem cells and demonstrate antagonistic roles in the regulation of cardiac differentiation. Journal of Tissue Engineering and Regenerative Medicine. 2017;11(3):787-99.
https://doi.org/10.1002/term.1977
PMid:25492026
Cao W, Shi P, Ge JJ. miR-21 enhances cardiac fibrotic remodeling and fibroblast proliferation via CADM1/STAT3 pathway. BMC Cardiovascular Disorders. 2017 Mar 23;17(1):88.
https://doi.org/10.1186/s12872-017-0520-7
PMid:28335740 PMCid:PMC5364650
van Rooij E, Sutherland LB, Thatcher JE, DiMaio JM, Naseem RH, Marshall WS, et al. Dysregulation of microRNAs after myocardial infarction reveals a role of miR-29 in cardiac fibrosis. Proc Natl Acad Sci U S A. 2008 Sep 2;105(35):13027-32.
https://doi.org/10.1073/pnas.0805038105
PMid:18723672 PMCid:PMC2529064
Turner NA, Porter KE. Function and fate of myofibroblasts after myocardial infarction. Fibrogenesis & Tissue Repair. 2013 Mar 1;6(1):5.
https://doi.org/10.1186/1755-1536-6-5
PMid:23448358 PMCid:PMC3599637
Wang G, Zhu J, Zhang J, Li Q, Li Y, He J, et al. Circulating microRNA: a novel potential biomarker for early diagnosis of acute myocardial infarction in humans. European heart journal. 2010;31(6):659-66.
https://doi.org/10.1093/eurheartj/ehq013
PMid:20159880
Xue S, Liu D, Zhu W, Su Z, Zhang L, Zhou C. Circulating miR-17-5p, miR-126-5p, and miR-145-3p Are Novel Biomarkers for Diagnosis of Acute Myocardial Infarction. Frontiers in Physiology. 2019;10:123.
https://doi.org/10.3389/fphys.2019.00123
PMid:30833907 PMCid:PMC6387945
Wang K, Zhao X, Liu Y, Zeng Q, Mao X, Li S, et al. Circulating MiR-19b-3p, MiR-134-5p and MiR-186-5p are Promising Novel Biomarkers for Early Diagnosis of Acute Myocardial Infarction. Cellular Physiology and Biochemistry. 2016;38:1015-29.
https://doi.org/10.1159/000443053
PMid:26939053
Venugopal P, Balakrishnan K, Kandadai SD, George M. Usefullness of MicroRNAs in Predicting the Clinical Outcome of Patients with Acute Myocardial Infarction During Follow-Up: A Systematic Review. Genetic testing and molecular biomarkers. 2022;26 5:277-89.
https://doi.org/10.1089/gtmb.2021.0283
PMid:35638909
Senmatsu S, Hirota K. Roles of lncRNA transcription as a novel regulator of chromosomal function. Genes Genet Syst. 2021 Feb 11;95(5):213-23.
https://doi.org/10.1266/ggs.20-00024
PMid:33028747
Niu X, Zhang J, Hu S, Dang W, Wang K, Bai M. lncRNA Oip5-as1 inhibits excessive mitochondrial fission in myocardial ischemia/reperfusion injury by modulating DRP1 phosphorylation. Cellular & Molecular Biology Letters. 2024 May 14;29(1):72.
https://doi.org/10.1186/s11658-024-00588-4
PMid:38745296 PMCid:PMC11092055
Du L, Chen J, Wu Y, Xia G, Chen M, Zhao P, et al. Long Non-coding RNA N1LR Protects Against Myocardial Ischemic/Reperfusion Injury Through Regulating the TGF-β Signaling Pathway. Front Cardiovasc Med. 2021 Aug 13;8:654969.
https://doi.org/10.3389/fcvm.2021.654969
PMid:34485393 PMCid:PMC8414635
Liu N, Kataoka M, Wang Y, Pu L, Dong X, Fu X, et al. LncRNA LncHrt preserves cardiac metabolic homeostasis and heart function by modulating the LKB1-AMPK signaling pathway. Basic Res Cardiol. 2021 Aug 11;116(1):48.
https://doi.org/10.1007/s00395-021-00887-3
PMid:34379189 PMCid:PMC8357683
Xu XZ, Luo B, Xiao Y, Zheng WQ. Effects of lncRNA MALAT1-mediated β-catenin signaling pathway on myocardial cell apoptosis in rats with myocardial ischemia/reperfusion injury. European Review for Medical and Pharmacological Sciences. 2019 Nov;23(21):9557-65.
Hu H, Wu J, Yu X, Zhou J, Yu H, Ma L. Long non-coding RNA MALAT1 enhances the apoptosis of cardiomyocytes through autophagy inhibition by regulating TSC2-mTOR signaling. Biological Research. 2019 Nov 29;52(1):58.
https://doi.org/10.1186/s40659-019-0265-0
PMid:31783925 PMCid:PMC6883637
Arslan S, Şahin NÖ, Bayyurt B, Berkan Ö, Yılmaz MB, Aşam M, et al. Role of lncRNAs in Remodeling of the Coronary Artery Plaques in Patients with Atherosclerosis. Mol Diagn Ther. 2023 Sep 1;27(5):601-10.
https://doi.org/10.1007/s40291-023-00659-w
PMid:37347334
Josefs T, Boon RA. The Long Non-coding Road to Atherosclerosis. Curr Atheroscler Rep. 2020 Aug 9;22(10):55.
https://doi.org/10.1007/s11883-020-00872-6
PMid:32772181 PMCid:PMC7415749
Li C, Sun J, Liu Q, Dodlapati S, Ming H, Wang L, et al. The landscape of accessible chromatin in quiescent cardiac fibroblasts and cardiac fibroblasts activated after myocardial infarction. Epigenetics. 2022 Sep 2;17(9):1020-39.
https://doi.org/10.1080/15592294.2021.1982158
PMid:34551670 PMCid:PMC9487753
Salvatori F, D'Aversa E, Serino ML, Singh AV, Secchiero P, Zauli G, et al. miRNAs Epigenetic Tuning of Wall Remodeling in the Early Phase after Myocardial Infarction: A Novel Epidrug Approach. IJMS. 2023 Aug 26;24(17):13268.
https://doi.org/10.3390/ijms241713268
PMid:37686073 PMCid:PMC10487654
Han P, Hang CT, Yang J, Chang CP. Chromatin Remodeling in Cardiovascular Development and Physiology. Circulation Research. 2011 Feb 4;108(3):378-96.
https://doi.org/10.1161/CIRCRESAHA.110.224287
PMid:21293009 PMCid:PMC3079363
Mathiyalagan P, Keating ST, Du XJ, El-Osta A. Chromatin modifications remodel cardiac gene expression. Cardiovascular Research. 2014 Jul 1;103(1):7-16.
https://doi.org/10.1093/cvr/cvu122
PMid:24812277
Takeuchi JK, Lou X, Alexander JM, Sugizaki H, Delgado-Olguín P, Holloway AK, et al. Chromatin remodelling complex dosage modulates transcription factor function in heart development. Nat Commun. 2011 Feb 8;2(1):187.
https://doi.org/10.1038/ncomms1187
PMid:21304516 PMCid:PMC3096875
Bieluszewski T, Prakash S, Roulé T, Wagner D. The Role and Activity of SWI/SNF Chromatin Remodelers. Annu Rev Plant Biol. 2023 May 22;74:139-63.
https://doi.org/10.1146/annurev-arplant-102820-093218
PMid:36889009
Su J, Li J, Yu Q, Wang J, Li X, Yang J, et al. Exosomal miRNAs as potential biomarkers for acute myocardial infarction. IUBMB Life. 2020;72(3):384-400.
https://doi.org/10.1002/iub.2189
PMid:31675148
Krolevets M, Cate VT, Prochaska JH, Schulz A, Rapp S, Tenzer S, et al. DNA methylation and cardiovascular disease in humans: a systematic review and database of known CpG methylation sites. Clin Epigenet. 2023 Mar 30;15(1):56.
https://doi.org/10.1186/s13148-023-01468-y
PMid:36991458 PMCid:PMC10061871
Qin M, Wu Q, Tian X, Zhu Q, Fang X, Chen X, et al. DNA Methylation Predicts Adverse Outcomes of Coronary Artery Disease . medRxiv. 2023;10(7) :23296703.
https://doi.org/10.1101/2023.10.07.23296703
Scărlătescu AI, Barbălată T, Sima AV, Stancu C, Niculescu L Ștefan, Micheu MM. miR-146a-5p, miR-223-3p and miR-142-3p as Potential Predictors of Major Adverse Cardiac Events in Young Patients with Acute ST Elevation Myocardial Infarction-Added Value over Left Ventricular Myocardial Work Indices. Diagnostics. 2022 Aug 12;12(8):1946.
https://doi.org/10.3390/diagnostics12081946
PMid:36010296 PMCid:PMC9406722
Zheng M, Liu X, Han R, Yuan W, Sun K, Zhong J, et al. Circulating exosomal long non‐coding RNAs in patients with acute myocardial infarction. J Cell Mol Med. 2020 Aug;24(16):9388-96.
https://doi.org/10.1111/jcmm.15589
PMid:32649009 PMCid:PMC7417690
Chen G, Huang S, Song F, Zhou Y, He X. Lnc-Ang362 is a pro-fibrotic long non-coding RNA promoting cardiac fibrosis after myocardial infarction by suppressing Smad7. Archives of Biochemistry and Biophysics. 2020 May;685:108354.
https://doi.org/10.1016/j.abb.2020.108354
PMid:32240638
Li Y. Modern epigenetics methods in biological research. Methods. 2021 Mar;187:104-13.
https://doi.org/10.1016/j.ymeth.2020.06.022
PMid:32645449 PMCid:PMC7785612
Stirzaker C, Song J, Davidson B, Clark S. Transcriptional Gene Silencing Promotes DNA Hypermethylation through a Sequential Change in Chromatin Modifications in Cancer Cells. Cancer Research. 2004;64:3871-7.
https://doi.org/10.1158/0008-5472.CAN-03-3690
PMid:15172996
Xu X, Elkenani M, Tan X, Hain JK, Cui B, Schnelle M, et al. DNA Methylation Analysis Identifies Novel Epigenetic Loci in Dilated Murine Heart upon Exposure to Volume Overload. IJMS. 2023 Mar 20;24(6):5885.
https://doi.org/10.3390/ijms24065885
PMid:36982963 PMCid:PMC10059258
Chiara LD, Leiro-Fernández V, Rodríguez-Girondo M, Valverde D, Botana-Rial M, Fernández-Villar A. Comparison of Bisulfite Pyrosequencing and Methylation-Specific qPCR for Methylation Assessment. International Journal of Molecular Sciences . 2020 Dec 3;21(23):9242.
https://doi.org/10.3390/ijms21239242
PMid:33287451 PMCid:PMC7730915
Kanuri SH, Ipe J, Kassab K, Gao H, Liu Y, Skaar TC, et al. Next generation MicroRNA sequencing to identify coronary artery disease patients at risk of recurrent myocardial infarction. Atherosclerosis. 2018 Nov;278:232-9.
https://doi.org/10.1016/j.atherosclerosis.2018.09.021
PMid:30336307 PMCid:PMC6350783
Papari E, Noruzinia M, Kashani L, Foster WG. Identification of candidate microRNA markers of endometriosis with the use of next-generation sequencing and quantitative real-time polymerase chain reaction. Fertility and Sterility. 2020 Jun;113(6):1232-41.
https://doi.org/10.1016/j.fertnstert.2020.01.026
PMid:32482255
Liu Y, Lu Q. Extracellular Vesicle MicroRNAs: Biomarker Discovery in Various Diseases Based on RT-QPCR. Biomarkers in Medicine. 2015 Aug 1;9(8):791-805.
https://doi.org/10.2217/BMM.15.45
PMid:26287938
Boštjančič E, Zidar N, Glavač D. MicroRNA microarray expression profiling in human myocardial infarction. Disease Markers. 2009 Jan 1;27(6):255-68.
https://doi.org/10.1155/2009/641082
PMid:20075508
Mehta J, Fox A, Pepper A, Mehta P. A promising target for novel therapies in cardiovascular disease. Journal of the American College of Cardiology. 2015;65:134-40.
Huang D, Cui L, Ahmed S, Zainab F, Wu Q, Wang X, et al. An overview of epigenetic agents and natural nutrition products targeting DNA methyltransferase, histone deacetylases and microRNAs. Food and chemical toxicology : an international journal published for the British Industrial Biological Research Association. 2019;123:574-94.
https://doi.org/10.1016/j.fct.2018.10.052
PMid:30408543
Saheli M, Pirhajati Mahabadi V, Mesbah-Namin SA, Seifalian A, Bagheri-Hosseinabadi Z. DNA methyltransferase inhibitor 5-azacytidine in high dose promotes ultrastructural maturation of cardiomyocyte. Stem Cell Investig. 2020 Dec 15;7:22.
https://doi.org/10.21037/sci-2020-007
PMid:33437842 PMCid:PMC7791156
Han W, Wang W, Wang Q, Maduray K, Hao L, Zhong J. A review on regulation of DNA methylation during post-myocardial infarction. Front Pharmacol. 2024 Feb 13;15:1267585.
https://doi.org/10.3389/fphar.2024.1267585
PMid:38414735 PMCid:PMC10896928
Chun P. Therapeutic effects of histone deacetylase inhibitors on heart disease. Arch Pharm Res. 2020 Dec 1;43(12):1276-96.
https://doi.org/10.1007/s12272-020-01297-0
PMid:33245518
Xie M, Kong Y, Tan W, May H, Battiprolu PK, Pedrozo Z, et al. Histone Deacetylase Inhibition Blunts Ischemia/Reperfusion Injury by Inducing Cardiomyocyte Autophagy. Circulation. 2014 Mar 11;129(10):1139-51.
https://doi.org/10.1161/CIRCULATIONAHA.113.002416
PMid:24396039 PMCid:PMC3984913
Granger A, Abdullah I, Huebner F, Stout A, Wang T, Huebner T, et al. Histone deacetylase inhibition reduces myocardial ischemia-reperfusion injury in mice. FASEB J. 2008 Oct;22(10):3549-60.
https://doi.org/10.1096/fj.08-108548
PMid:18606865 PMCid:PMC2537432
Kowara M, Borodzicz-Jazdzyk S, Rybak K, Kubik M, Cudnoch-Jedrzejewska A. Therapies Targeted at Non-Coding RNAs in Prevention and Limitation of Myocardial Infarction and Subsequent Cardiac Remodeling-Current Experience and Perspectives. International Journal of Molecular Sciences. 2021 Jan;22(11):5718.
https://doi.org/10.3390/ijms22115718
PMid:34071976 PMCid:PMC8198996
Liu N, Bezprozvannaya S, Williams AH, Qi X, Richardson JA, Bassel-Duby R, et al. microRNA-133a regulates cardiomyocyte proliferation and suppresses smooth muscle gene expression in the heart. Genes Dev. 2008 Dec 1;22(23):3242-54.
https://doi.org/10.1101/gad.1738708
PMid:19015276 PMCid:PMC2600761
Yang L, Wang B, Zhou Q, Wang Y, Liu X, Liu Z, et al. MicroRNA-21 prevents excessive inflammation and cardiac dysfunction after myocardial infarction through targeting KBTBD7. Cell Death Dis. 2018 Jul 10;9(7):1-14.
https://doi.org/10.1038/s41419-018-0805-5
PMid:29991775 PMCid:PMC6039462
O'Donoghue ML, Rosenson RS, Gencer B, López JAG, Lepor NE, Baum SJ, et al. Small Interfering RNA to Reduce Lipoprotein(a) in Cardiovascular Disease. New England Journal of Medicine. 2022 Nov 16;387(20):1855-64.
https://doi.org/10.1056/NEJMoa2211023
PMid:36342163
Koren MJ, Moriarty PM, Baum SJ, Neutel J, Hernandez-Illas M, Weintraub HS, et al. Preclinical development and phase 1 trial of a novel siRNA targeting lipoprotein(a). Nat Med. 2022 Jan;28(1):96-103.
https://doi.org/10.1038/s41591-021-01634-w
PMid:35027752
Deng J, Guo M, Li G, Xiao J. Gene therapy for cardiovascular diseases in China: basic research. Gene Ther. 2020 Aug;27(7):360-9.
https://doi.org/10.1038/s41434-020-0148-6
PMid:32341485
Li G, Zhang X, Wang H, Liu D, Li Z, Wu Z, et al. Increasing CRISPR/Cas9-mediated homology-directed DNA repair by histone deacetylase inhibitors. The international journal of biochemistry & cell biology. 2020 Aug 1;125:105790.
https://doi.org/10.1016/j.biocel.2020.105790
PMid:32534122
Schary Y, Rotem I, Caller T, Lewis N, Shaihov-Teper O, Brzezinski RY, et al. CRISPR-Cas9 editing of TLR4 to improve the outcome of cardiac cell therapy. Sci Rep. 2023 Mar 18;13(1):4481.
https://doi.org/10.1038/s41598-023-31286-4
PMid:36934130 PMCid:PMC10024743
Sheikh AQ, Chau BM, Druckman ME, Khatri P. Epigenetic regulation of cardiovascular disease-recent advances and future perspectives. Cardiovascular research. 2016;109:17-28.
Schiano C, Vietri M, Grimaldi V, Picascia A, De Pascale MD, Napoli C. Epigenetic-related therapeutic challenges in cardiovascular disease. Trends in pharmacological sciences. 2015;36:226-35.
https://doi.org/10.1016/j.tips.2015.02.005
PMid:25758254
Sahoo S, Kariya T, Ishikawa K. Targeted delivery of therapeutic agents to the heart. Nat Rev Cardiol. 2021 Jun;18(6):389-99.
https://doi.org/10.1038/s41569-020-00499-9
PMid:33500578 PMCid:PMC8140998
Xue X, Shi X, Dong H, You S, Cao H, Wang K, Wen Y, Shi D, He B, Li Y. Delivery of microRNA-1 inhibitor by dendrimer-based nanovector: An early targeting therapy for myocardial infarction in mice. Nanomedicine: Nanotechnology, Biology and Medicine. 2018 Feb 1;14(2):619-31.
https://doi.org/10.1016/j.nano.2017.12.004
PMid:29269324
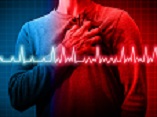
Published
How to Cite
Issue
Section
License
Copyright (c) 2024 Galen Medical Journal

This work is licensed under a Creative Commons Attribution 4.0 International License.