Dissecting Uricase Immunogenicity: Unveiling the Role of Quaternary Epitopes through In Silico Analysis
Quaternary Epitope Insights in Uricase Immunogenicity
DOI:
https://doi.org/10.31661/gmj.v14i.3634Keywords:
Immunogenicity; Quaternary Epitopes; Epitope Mapping; In Silico Analysis; Computational Immunology; Therapeutic ProteinsAbstract
Background: Protein-based therapeutics offer remarkable precision and effectiveness, yet their immunogenic potential remains a significant challenge. Uricase, an enzyme used to treat hyperuricemia, is no exception, often eliciting immune responses due to its non-human origins and repeated administration requirements. Understanding the immunogenic mechanisms at play is crucial for enhancing therapeutic efficacy. Materials and Methods: This in silico study investigates the immunogenic landscape of uricase, focusing on the identification of linear, conformational, and the underexplored quaternary epitopes. Using a comprehensive approach, we analyzed multiple uricase variants through structural alignments, epitope prediction algorithms, and network-based residue interaction models. Predictive tools, including BepiPred, DiscoTope, and SEMA, were employed to identify epitope regions, with a novel focus on quaternary epitopes formed by inter-chain interactions. Results: Our analysis reveals conserved structural motifs across uricase variants, with linear and conformational epitopes localized in similar regions. The groundbreaking identification of quaternary epitopes—epitopes formed through interactions between protein chains—provides a novel insight into uricase immunogenicity. These epitopes, located in structurally prominent regions, likely play a critical role in the immune response to uricase. Conclusions: This study marks a significant advance in understanding uricase immunogenicity, introducing quaternary epitopes as pivotal factors in immune recognition. The findings open new avenues for designing uricase variants with reduced immunogenicity, offering potential improvements in therapeutic strategies for hyperuricemia management.
References
Douthwaite J, Jermutus L. Exploiting directed evolution for the discovery of biologicals. Current opinion in drug discovery & development. 2006;9(2):269-75.
Qin X, Yu C, Wei J, Li L, Zhang C, Wu Q et al. Rational design of nanocarriers for intracellular protein delivery. Advanced Materials. 2019;31(46):1902791.
https://doi.org/10.1002/adma.201902791
PMid:31496027
Bandyopadhyay A. Complexities of protein therapeutics and immunogenicity. Journal of Bioanalysis & Biomedicine. 2015;7(3):70-4.
https://doi.org/10.4172/1948-593X.1000126
De Groot AS, Scott DW. Immunogenicity of protein therapeutics. Trends Immunol. 2007;28(11):482-90.
https://doi.org/10.1016/j.it.2007.07.011
PMid:17964218
Fogdell‐Hahn A. Antidrug antibodies: B cell immunity against therapy. Scand J Immunol. 2015;82(3):184-90.
https://doi.org/10.1111/sji.12327
PMid:26098690
Enrico D, Paci A, Chaput N, Karamouza E, Besse B. Antidrug antibodies against immune checkpoint blockers: impairment of drug efficacy or indication of immune activation? Clin Cancer Res. 2020;26(4):787-92.
https://doi.org/10.1158/1078-0432.CCR-19-2337
PMid:31757876
Cheng X, Changgui L. The principles of gout therapy. Gout Hyperuricemia. 2015;2(1):15-23.
Schlesinger N. Hyperuricemia, gout, and diet. Nutrition and Rheumatic Disease. 2008:169-82.
https://doi.org/10.1007/978-1-59745-403-2_10
Sherman MR, Saifer MG, Perez-Ruiz F. PEG-uricase in the management of treatment-resistant gout and hyperuricemia. Advanced drug delivery reviews. 2008;60(1):59-68.
https://doi.org/10.1016/j.addr.2007.06.011
PMid:17826865
Colloc'h N, Hajji ME, Bachet B, l'Hermite G, Schiltz M, Prangé T et al. Crystal structure of the protein drug urate oxidase-inhibitor complex at 2.05 Å resolution. Nat Struct Biol. 1997;4(11):947-52.
https://doi.org/10.1038/nsb1197-947
PMid:9360612
Terkeltaub R. Update on gout: new therapeutic strategies and options. Nature Reviews Rheumatology. 2010;6(1):30.
https://doi.org/10.1038/nrrheum.2009.236
PMid:20046204
Khaniukov O, Chornyi V, Yevstihnieiev I, Hutnik I, Smolianova O, Romuz N, et al. LESCH-NYHAN SYNDROME - late diagnosis of rare disease: clinical case. The Journal of V N Karazin Kharkiv National University, series & quot Medicine& quot. 2021;0(41):e.
Li L, Zhang Y, Zeng C. Update on the epidemiology, genetics, and therapeutic options of hyperuricemia. Am J Transl Res. 2020;12(7):3167-81.
Nyborg AC, Ward C, Zacco A, Chacko B, Grinberg L, Geoghegan JC et al. A Therapeutic Uricase with Reduced Immunogenicity Risk and Improved Development Properties. PLoS One. 2016;11(12):e0167935.
https://doi.org/10.1371/journal.pone.0167935
PMid:28002433 PMCid:PMC5176304
Yang X, Yuan Y, Zhan CG, Liao F. Uricases as therapeutic agents to treat refractory gout: Current states and future directions. Drug development research. 2012;73(2):66-72.
https://doi.org/10.1002/ddr.20493
PMid:22665944 PMCid:PMC3365602
Sands E, Kivitz A, DeHaan W, et al. P109 Clinical development of a novel strategy to mitigate biologic immunogenicity: monthly dosing of a pegylated uricase with SVP-R enables sustained reduction of serum uric acid (SUA) levels by mitigating formation of anti-drug antibodies (ADAS)Annals of the Rheumatic Diseases. BMJ Publishing Group Ltd. 2018;77:A59-A60.
https://doi.org/10.1136/annrheumdis-2018-EWRR2018.124
Nelapati AK, Das BK, Ettiyappan JBP, Chakraborty D. In-silico epitope identification and design of Uricase mutein with reduced immunogenicity. Process Biochemistry. 2020;92:288-302.
https://doi.org/10.1016/j.procbio.2020.01.022
Van Regenmortel MH. Protein structure and antigenicity. International Journal of Radiation Applications and Instrumentation Part B Nuclear Medicine and Biology. 1987;14(4):277-80.
https://doi.org/10.1016/0883-2897(87)90003-1
PMid:2443466
Onda M. Reducing the immunogenicity of protein therapeutics. Curr Drug Targets. 2009;10(2):131-9.
https://doi.org/10.2174/138945009787354511
PMid:19199909
Hrabe T, Li Z, Sedova M, Rotkiewicz P, Jaroszewski L, Godzik A. PDBFlex: exploring flexibility in protein structures. Nucleic Acids Res. 2016;44(D1):D423-D8.
https://doi.org/10.1093/nar/gkv1316
PMid:26615193 PMCid:PMC4702920
Pettersen EF, Goddard TD, Huang CC, Couch GS, Greenblatt DM, Meng EC et al. UCSF Chimera-a visualization system for exploratory research and analysis. J Comput Chem. 2004;25(13):1605-12.
https://doi.org/10.1002/jcc.20084
PMid:15264254
Waterman MS, Eggert M. A new algorithm for best subsequence alignments with application to tRNA-rRNA comparisons. J Mol Biol. 1987;197(4):723-8.
https://doi.org/10.1016/0022-2836(87)90478-5
PMid:2448477
Parker J, Guo D, Hodges R. New hydrophilicity scale derived from high-performance liquid chromatography peptide retention data: correlation of predicted surface residues with antigenicity and X-ray-derived accessible sites. Biochemistry. 1986;25(19):5425-32.
https://doi.org/10.1021/bi00367a013
PMid:2430611
Karplus P, Schulz G. Prediction of chain flexibility in proteins. Naturwissenschaften. 1985;72(4):212-3.
https://doi.org/10.1007/BF01195768
Emini EA, Hughes JV, Perlow D, Boger J. Induction of hepatitis A virus-neutralizing antibody by a virus-specific synthetic peptide. J Virol. 1985;55(3):836-9.
https://doi.org/10.1128/jvi.55.3.836-839.1985
PMid:2991600 PMCid:PMC255070
Kolaskar AS, Tongaonkar PC. A semi-empirical method for prediction of antigenic determinants on protein antigens. FEBS letters. 1990 Dec 10;276(1-2):172-4.
https://doi.org/10.1016/0014-5793(90)80535-Q
PMid:1702393
Larsen JE, Lund O, Nielsen M. Improved method for predicting linear B-cell epitopes. Immunome Res. 2006;2:2.
https://doi.org/10.1186/1745-7580-2-2
PMid:16635264 PMCid:PMC1479323
Ponomarenko JV, Bourne PE. Antibody-protein interactions: benchmark datasets and prediction tools evaluation. BMC Struct Biol. 2007;7:64.
https://doi.org/10.1186/1472-6807-7-64
PMid:17910770 PMCid:PMC2174481
Haste Andersen P, Nielsen M, Lund O. Prediction of residues in discontinuous B-cell epitopes using protein 3D structures. Protein Sci. 2006;15(11):2558-67.
https://doi.org/10.1110/ps.062405906
PMid:17001032 PMCid:PMC2242418
Jespersen MC, Peters B, Nielsen M, Marcatili P. BepiPred-2.0: improving sequence-based B-cell epitope prediction using conformational epitopes. Nucleic acids research. 2017 Jul 3;45(W1):W24-9.
https://doi.org/10.1093/nar/gkx346
PMid:28472356 PMCid:PMC5570230
Clifford JN, Høie MH, Deleuran S, Peters B, Nielsen M, Marcatili P. BepiPred‐3.0: Improved B‐cell epitope prediction using protein language models. Protein Sci. 2022;31(12):e4497.
https://doi.org/10.1002/pro.4497
PMid:36366745 PMCid:PMC9679979
Ponomarenko J, Bui H-H, Li W, Fusseder N, Bourne PE, Sette A et al. ElliPro: a new structure-based tool for the prediction of antibody epitopes. BMC Bioinformatics. 2008;9(1):514.
https://doi.org/10.1186/1471-2105-9-514
PMid:19055730 PMCid:PMC2607291
Høie MH, Gade FS, Johansen JM, Würtzen C, Winther O, Nielsen M, Marcatili P. DiscoTope-3.0: improved B-cell epitope prediction using inverse folding latent representations. Frontiers in Immunology. 2024 Feb 8;15:1322712.
https://doi.org/10.3389/fimmu.2024.1322712
PMid:38390326 PMCid:PMC10882062
Shashkova TI, Umerenkov D, Salnikov M, Strashnov PV, Konstantinova AV, Lebed I et al. SEMA: Antigen B-cell conformational epitope prediction using deep transfer learning. Front Immunol. 2022:5272.
https://doi.org/10.1101/2022.06.20.496780
Doncheva NT, Klein K, Domingues FS, Albrecht M. Analyzing and visualizing residue networks of protein structures. Trends Biochem Sci. 2011;36(4):179-82.
https://doi.org/10.1016/j.tibs.2011.01.002
PMid:21345680
Shannon P, Markiel A, Ozier O, Baliga NS, Wang JT, Ramage D et al. Cytoscape: a software environment for integrated models of biomolecular interaction networks. Genome Res. 2003;13(11):2498-504.
https://doi.org/10.1101/gr.1239303
PMid:14597658 PMCid:PMC403769
Leader B, Baca QJ, Golan DE. Protein therapeutics: a summary and pharmacological classification. Nature reviews Drug discovery. 2008;7(1):21-39.
https://doi.org/10.1038/nrd2399
PMid:18097458
Tsukada H, Mochizuki Y, Fujiwara S. The nucleoids of rat liver cell microbodies: fine structure and enzymes. The Journal of Cell Biology. 1966;28(3):449-60.
https://doi.org/10.1083/jcb.28.3.449
PMid:5960808 PMCid:PMC2106947
Burns CM, Wortmann RL. Gout therapeutics: new drugs for an old disease. The Lancet. 2011;377(9760):165-77.
https://doi.org/10.1016/S0140-6736(10)60665-4
PMid:20719377
Hershfield MS, Roberts LJ, Ganson NJ, Kelly SJ, Santisteban I, Scarlett E et al. Treating gout with pegloticase, a PEGylated urate oxidase, provides insight into the importance of uric acid as an antioxidant in vivo. Proceedings of the National Academy of Sciences. 2010;107(32):14351-6.
https://doi.org/10.1073/pnas.1001072107
PMid:20660758 PMCid:PMC2922538
Wang X, Lu H, Rong J, Sun Z, Zheng Y, Fan B, Jia Z. PEGylated porcine-human recombinant uricase: A novel fusion protein with improved efficacy and safety for the treatment of hyperuricemia and renal complications. Open Life Sciences. 2024 Jan 23;19(1):20220799.
https://doi.org/10.1515/biol-2022-0799
PMid:38283118 PMCid:PMC10811527
Lipsky PE, Calabrese LH, Kavanaugh A, Sundy JS, Wright D, Wolfson M et al. Pegloticase immunogenicity: the relationship between efficacy and antibody development in patients treated for refractory chronic gout. Arthritis Res Ther. 2014;16:1-8.
https://doi.org/10.1186/ar4497
PMid:24588936 PMCid:PMC4060440
Khanna PP, Khanna D, Cutter G, Foster J, Melnick J, Jaafar S et al. Reducing immunogenicity of pegloticase with concomitant use of mycophenolate mofetil in patients with refractory gout: a phase II, randomized, double‐blind, placebo‐controlled trial. Arthritis & Rheumatology. 2021;73(8):1523-32.
https://doi.org/10.1002/art.41731
PMid:33750034 PMCid:PMC8324571
Guttmann A, Krasnokutsky S, Pillinger MH, Berhanu A. Pegloticase in gout treatment-safety issues, latest evidence and clinical considerations. Therapeutic advances in drug safety. 2017;8(12):379-88.
https://doi.org/10.1177/2042098617727714
PMid:29204266 PMCid:PMC5703101
Tripathi S, Parmar J, Kumar A. Structure-based immunogenicity prediction of uricase from fungal (Aspergillus flavus), bacterial (Bacillus subtillis) and mammalian sources using immunoinformatic approach. The Protein Journal. 2020 Apr;39:133-44.
https://doi.org/10.1007/s10930-020-09886-0
PMid:32221804
Sundy JS, Hershfield MS. Uricase and other novel agents for the management of patients with treatment-failure gout. Curr Rheumatol Rep. 2007;9(3):258-64.
https://doi.org/10.1007/s11926-007-0041-y
PMid:17531181
Sun J, Xu T, Wang S, Li G, Wu D, Cao Z. Does difference exist between epitope and non-epitope residues?. Immunome research. 2011 Sep 1;7(3):1.
Zhao L, Li J. Mining for the antibody-antigen interacting associations that predict the B cell epitopes. BMC structural biology. 2010;10(1):S6.
https://doi.org/10.1186/1472-6807-10-S1-S6
PMid:20487513 PMCid:PMC2873829
Wang Y, Wu W, Negre NN, White KP, Li C, Shah PK. Determinants of antigenicity and specificity in immune response for protein sequences. BMC bioinformatics. 2011;12(1):251.
https://doi.org/10.1186/1471-2105-12-251
PMid:21693021 PMCid:PMC3133554
Kringelum JV, Lundegaard C, Lund O, Nielsen M. Reliable B cell epitope predictions: impacts of method development and improved benchmarking. PLoS Comput Biol. 2012;8:e1002829.
https://doi.org/10.1371/journal.pcbi.1002829
PMid:23300419 PMCid:PMC3531324
Xu X, Sun J, Liu Q, Wang X, Xu T, Zhu R et al. Evaluation of spatial epitope computational tools based on experimentally-confirmed dataset for protein antigens. Chinese Science Bulletin. 2010;55(20):2169-74.
https://doi.org/10.1007/s11434-010-3199-z
Yao B, Zheng D, Liang S, Zhang C. Conformational B-cell epitope prediction on antigen protein structures: a review of current algorithms and comparison with common binding site prediction methods. PLoS One. 2013;8(4):e62249.
https://doi.org/10.1371/journal.pone.0062249
PMid:23620816 PMCid:PMC3631208
ANAND KUMAR N, Kamala MS. A computational investigation on immunogenicity of uricase from aspergillus flavus and candida utilis. Physical Chemistry Research. 2023;11(3):683-93.
Tripathi S, Parmar J, Kumar A. Structure-based immunogenicity prediction of uricase from fungal (Aspergillus flavus), bacterial (Bacillus subtillis) and mammalian sources using immunoinformatic approach. The Protein Journal. 2020;39:133-44.
https://doi.org/10.1007/s10930-020-09886-0
PMid:32221804
Lo YT, Shih TC, Pai TW, Ho LP, Wu JL, Chou HY. Conformational epitope matching and prediction based on protein surface spiral features. BMC Genomics. 2021;22(Suppl 2):116.
https://doi.org/10.1186/s12864-020-07303-5
PMid:34058977 PMCid:PMC8165135
Gorny MK, Stamatatos L, Volsky B, Revesz K, Williams C, Wang X-H et al. Identification of a new quaternary neutralizing epitope on human immunodeficiency virus type 1 virus particles. J Virol. 2005;79(8):5232-7.
https://doi.org/10.1128/JVI.79.8.5232-5237.2005
PMid:15795308 PMCid:PMC1069558
Gilman MS, Moin SM, Mas V, Chen M, Patel NK, Kramer K et al. Characterization of a prefusion-specific antibody that recognizes a quaternary, cleavage-dependent epitope on the RSV fusion glycoprotein. PLoS Pathog. 2015;11(7):e1005035.
https://doi.org/10.1371/journal.ppat.1005035
PMid:26161532 PMCid:PMC4498696
Mittler E, Wec AZ, Tynell J, Guardado-Calvo P, Wigren-Byström J, Polanco LC et al. Human antibody recognizing a quaternary epitope in the Puumala virus glycoprotein provides broad protection against orthohantaviruses. Sci Transl Med. 2022;14(636):eabl5399.
https://doi.org/10.1126/scitranslmed.abl5399
PMid:35294259 PMCid:PMC9805701
Gallichotte EN, Baric TJ, Yount Jr BL, Widman DG, Durbin A, Whitehead S et al. Human dengue virus serotype 2 neutralizing antibodies target two distinct quaternary epitopes. PLoS Pathog. 2018;14(2):e1006934.
https://doi.org/10.1371/journal.ppat.1006934
PMid:29481552 PMCid:PMC5843351
Long F, Doyle M, Fernandez E, Miller AS, Klose T, Sevvana M et al. Structural basis of a potent human monoclonal antibody against Zika virus targeting a quaternary epitope. Proceedings of the National Academy of Sciences. 2019;116(5):1591-6.
https://doi.org/10.1073/pnas.1815432116
PMid:30642974 PMCid:PMC6358714
Tharakaraman K, Watanabe S, Chan KR, Huan J, Subramanian V, Chionh YH, Raguram A, Quinlan D, McBee M, Ong EZ, Gan ES. Rational engineering and characterization of an mAb that neutralizes Zika virus by targeting a mutationally constrained quaternary epitope. Cell host & microbe. 2018 May 9;23(5):618-27.
https://doi.org/10.1016/j.chom.2018.04.004
PMid:29746833 PMCid:PMC6018055
Crooks ET, Tong T, Chakrabarti B, Narayan K, Georgiev IS, Menis S et al. Vaccine-elicited tier 2 HIV-1 neutralizing antibodies bind to quaternary epitopes involving glycan-deficient patches proximal to the CD4 binding site. PLoS Pathog. 2015;11(5):e1004932.
https://doi.org/10.1371/journal.ppat.1004932
PMid:26023780 PMCid:PMC4449185
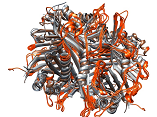
Published
How to Cite
Issue
Section
License
Copyright (c) 2025 Galen Medical Journal

This work is licensed under a Creative Commons Attribution 4.0 International License.