The Regulation of Pyroptosis and Ferroptosis by MicroRNAs in Cardiovascular Diseases
Pyroptosis and Ferroptosis Regulation by Micro RNAs
DOI:
https://doi.org/10.31661/gmj.v12i.2933Keywords:
Ferroptosis, Pyroptosis, MiRNAs, Cardiovascular Diseases, Heart FailureAbstract
Cardiovascular diseases (CVDs) are considered the most prevalent noncommunicable disease and the leading cause of death worldwide. A plethora of evidence has revealed that microRNAs (miRNAs) could control the inhibition or progression of CVDs by regulating pivotal cell pro-cesses ranging from metabolism and homeostasis to programmed cell death (PCD). Pyroptosis and ferroptosis are two major types of nonapoptotic PCDs involved in the pathogenesis of heart failure. However, no study has discussed the crosstalk between miRNAs and these two types of PCDs in the CVDs. The current review demonstrated that different types of miRNAs can regu-late both ferroptosis and pyroptosis and thereby affect CVDs progression and inhibition. Alto-gether, the discussed content encourages further studies to confirm that mentioned pathways are suitable to be considered as novel therapeutic approaches against CVDs.
References
Tabrizi MT, Khezerlu N, Rabori VS, Sarvestani AH. The assessment of functional indices of left ventricular wall layers in cases with normal and high blood pressure by layer-specific strain methods. J Res Clin Med. 2022;10:7.
https://doi.org/10.34172/jrcm.2022.007
Raberi VS, Ezati E, Zadeh RF. The relationship between the hematologic indices (PDW, WBC count, MPV) at the admission time and descending ST segment after thrombolysis in patients with myocardial infarction. Amazon Investig. 2019;8(18):139-49.
Amini M, Zayeri F, Salehi M. Trend analysis of cardiovascular disease mortality, incidence, and mortality-to-incidence ratio: results from global burden of disease study 2017. BMC Public Health. 2021;21(1):1-12.
https://doi.org/10.1186/s12889-021-10429-0
Şahin B, İlgün G. Risk factors of deaths related to cardiovascular diseases in World Health Organization (WHO) member countries. Health Soc Care Community. 2022;30(1):73-80.
https://doi.org/10.1111/hsc.13156
Vatani KK, Raberi VS, Khalili N, Ajdari S. The Association Between the Serum Level of 25-Hydroxy Vitamin D and the Echocardiographic Indices of Left Ventricular Function in Patients With no Significant Coronary Artery Disease. Hypertension. 2020;45:220-4.
Tabrizi MT, Khezerlouy-Aghdam N, Raberi VS, Khosroshahi AJ. Aortic shelf as a normal variant diagnosed primarily as the aortic dissection: A case report. J Cardiovasc Thorac Res. 2020;12(3):234.
https://doi.org/10.34172/jcvtr.2020.41
Groenewegen A, Rutten FH, Mosterd A, Hoes AW. Epidemiology of heart failure. Eur J Heart Fail. 2020;22(8):1342-56.
https://doi.org/10.1002/ejhf.1858
Braunwald E. The war against heart failure: the Lancet lecture. The Lancet. 2015;385(9970):812-24.
https://doi.org/10.1016/S0140-6736(14)61889-4
Truby LK, Rogers JG. Advanced heart failure: epidemiology, diagnosis, and therapeutic approaches. Heart Fail. 2020;8(7):523-36.
https://doi.org/10.1016/j.jchf.2020.01.014
Lee H, Zhang Z, Krause HM. Long noncoding RNAs and repetitive elements: junk or intimate evolutionary partners? Trends Genet. 2019;35(12):892-902.
https://doi.org/10.1016/j.tig.2019.09.006
Abbas N, Perbellini F, Thum T. Non-coding RNAs: emerging players in cardiomyocyte proliferation and cardiac regeneration. Basic Res Cardiol. 2020;115(5):1-20.
https://doi.org/10.1007/s00395-020-0816-0
Xu Z, Yan Y, Qian L, Gong Z. Long non-coding RNAs act as regulators of cell autophagy in diseases. Oncol Rep. 2017;37(3):1359-66.
https://doi.org/10.3892/or.2017.5416
Zhu L, Li N, Sun L, Zheng D, Shao G. Non-coding RNAs: The key detectors and regulators in cardiovascular disease. Genomics. 2021;113(1):1233-46.
https://doi.org/10.1016/j.ygeno.2020.10.024
Beylerli O, Gareev I, Sufianov A, Ilyasova T, Guang Y. The role of microRNA in the pathogenesis of glial brain tumors. Non-coding RNA Res. 2022;7(2):71-6.
https://doi.org/10.1016/j.ncrna.2022.02.005
Lee RC, Feinbaum RL, Ambros V. The C elegans heterochronic gene lin-4 encodes small RNAs with antisense complementarity to lin-14. Cell. 1993;75(5):843-54.
https://doi.org/10.1016/0092-8674(93)90529-Y
Wightman B, Ha I, Ruvkun G. Posttranscriptional regulation of the heterochronic gene lin-14 by lin-4 mediates temporal pattern formation in C. elegans Cell. 1993;75(5):855-62.
https://doi.org/10.1016/0092-8674(93)90530-4
Pajares MJ, Alemany-Cosme E, Goñi S, Bandres E, Palanca-Ballester C, Sandoval J. Epigenetic regulation of microRNAs in cancer: shortening the distance from bench to bedside. Int J Mol Sci. 2021;22(14):7350.
https://doi.org/10.3390/ijms22147350
Xiao M, Li J, Li W, Wang Y, Wu F, Xi Y et al. MicroRNAs activate gene transcription epigenetically as an enhancer trigger. RNA Biol. 2017;14(10):1326-34.
https://doi.org/10.1080/15476286.2015.1112487
O'Brien J, Hayder H, Zayed Y, Peng C. Overview of microRNA biogenesis, mechanisms of actions, and circulation. Front Endocrinol (Lausanne). 2018;9:402.
https://doi.org/10.3389/fendo.2018.00402
Pandita D. Role of miRNA technology and miRNAs in abiotic and biotic stress resilience. Plant Perspectives to Global Climate Changes. Elsevier; 2022:303-30.
https://doi.org/10.1016/B978-0-323-85665-2.00015-7
Mayya VK. Understanding molecular mechanisms of microRNA-mediated gene silencing. McGill University: Canada; 2021.
-Huang V. Endogenous miRNAa: miRNA-mediated gene upregulation. RNA Activation. 2017:65-79.
https://doi.org/10.1007/978-981-10-4310-9_5
Desantis V, Solimando AG, Saltarella I, Sacco A, Giustini V, Bento M et al. MicroRNAs as a potential new preventive approach in the transition from asymptomatic to symptomatic multiple myeloma disease. Cancers (Basel). 2021;13(15):3650.
https://doi.org/10.3390/cancers13153650
Makarova J, Turchinovich A, Shkurnikov M, Tonevitsky A. Extracellular miRNAs and cell-cell communication: problems and prospects. Trends Biochem Sci. 2021;46(8):640-51.
https://doi.org/10.1016/j.tibs.2021.01.007
Schulte C, Westermann D, Blankenberg S, Zeller T. Diagnostic and prognostic value of circulating microRNAs in heart failure with preserved and reduced ejection fraction. World J Cardiol. 2015;7(12):843.
https://doi.org/10.4330/wjc.v7.i12.843
Schulte C, Zeller T. microRNA-based diagnostics and therapy in cardiovascular disease-Summing up the facts. Cardiovasc Diagn Ther. 2015;5(1):17.
Zampetaki A, Mayr M. MicroRNAs in vascular and metabolic disease. Circ Res. 2012;110(3):508-22.
https://doi.org/10.1161/CIRCRESAHA.111.247445
Menghini R, Stöhr R, Federici M. MicroRNAs in vascular aging and atherosclerosis. Ageing res rev. 2014;17:68-78.
https://doi.org/10.1016/j.arr.2014.03.005
Anand S. A brief primer on microRNAs and their roles in angiogenesis. Vascular cell. 2013;5(1):1-7.
https://doi.org/10.1186/2045-824X-5-2
Schulte C, Karakas M, Zeller T. microRNAs in cardiovascular disease-clinical application. Clin Chem Lab Med. 2017;55(5):687-704.
https://doi.org/10.1515/cclm-2016-0576
Schulte C, Mayr M. MicroRNAs: a new understanding of platelet physiology and pathology. Thromb Haemost. 2019;119(02):191-.
https://doi.org/10.1055/s-0038-1677505
Dong S, Cheng Y, Yang J, Li J, Liu X, Wang X et al. MicroRNA expression signature and the role of microRNA-21 in the early phase of acute myocardial infarction. J Biol Chem. 2009;284(43):29514-25.
https://doi.org/10.1074/jbc.M109.027896
Ye Y, Perez-Polo JR, Qian J, Birnbaum Y. The role of microRNA in modulating myocardial ischemia-reperfusion injury. Physiol Genomics. 2011;43(10):534-42.
https://doi.org/10.1152/physiolgenomics.00130.2010
Barwari T, Joshi A, Mayr M. MicroRNAs in cardiovascular disease. J Am Coll Cardiol. 2016;68(23):2577-84.
https://doi.org/10.1016/j.jacc.2016.09.945
Care A, Catalucci D, Felicetti F, Bonci D, Addario A, Gallo P et al. MicroRNA-133 controls cardiac hypertrophy. Nat Med. 2007;13(5):613-8.
https://doi.org/10.1038/nm1582
Elia L, Contu R, Quintavalle M, Varrone F, Chimenti C, Russo MA et al. Reciprocal regulation of microRNA-1 and insulin-like growth factor-1 signal transduction cascade in cardiac and skeletal muscle in physiological and pathological conditions. Circulation. 2009;120(23):2377-85.
https://doi.org/10.1161/CIRCULATIONAHA.109.879429
Zhao Y, Ransom JF, Li A, Vedantham V, von Drehle M, Muth AN et al. Dysregulation of cardiogenesis, cardiac conduction, and cell cycle in mice lacking miRNA-1-2. Cell. 2007;129(2):303-17.
https://doi.org/10.1016/j.cell.2007.03.030
Krenz M, Robbins J. Impact of beta-myosin heavy chain expression on cardiac function during stress. J Am Coll Cardiol. 2004;44(12):2390-7.
https://doi.org/10.1016/j.jacc.2004.09.044
Oh JG, Jang SP, Yoo J, Lee M-A, Lee SH, Lim T et al. Role of the PRC2-Six1-miR-25 signaling axis in heart failure. J Mol Cell Cardiol. 2019;129:58-68.
https://doi.org/10.1016/j.yjmcc.2019.01.017
Jiang N, Zhang X, Gu X, Li X, Shang L. Progress in understanding the role of lncRNA in programmed cell death. Cell death discov. 2021;7(1):1-11.
https://doi.org/10.1038/s41420-021-00407-1
Peng F, Liao M, Qin R, Zhu S, Peng C, Fu L et al. Regulated cell death (RCD) in cancer: key pathways and targeted therapies. Signal Transduct Target Ther. 2022;7(1):1-66.
https://doi.org/10.1038/s41392-022-01110-y
Ruan J, Wang S, Wang J. Mechanism and regulation of pyroptosis-mediated in cancer cell death. Chem Biol Interact. 2020;323:109052.
https://doi.org/10.1016/j.cbi.2020.109052
Tang Y, Wang Y, Park K-m, Hu Q, Teoh J-p, Broskova Z et al. MicroRNA-150 protects the mouse heart from ischaemic injury by regulating cell death. Cardiovasc Res. 2015;106(3):387-97.
https://doi.org/10.1093/cvr/cvv121
Su M, Wang J, Wang C, Wang X, Dong W, Qiu W et al. MicroRNA-221 inhibits autophagy and promotes heart failure by modulating the p27/CDK2/mTOR axis. Cell Death Differ. 2015;22(6):986-99.
https://doi.org/10.1038/cdd.2014.187
Lai L, Chen J, Wang N, Zhu G, Duan X, Ling F. MiRNA-30e mediated cardioprotection of ACE2 in rats with Doxorubicin-induced heart failure through inhibiting cardiomyocytes autophagy. Life sciences. 2017;169:69-75.
https://doi.org/10.1016/j.lfs.2016.09.006
Liu Z, Wang M, Cheng A, Ou X, Mao S, Yang Q et al. Gene regulation in animal miRNA biogenesis. Epigenomics. 2022;14(19):1197-212.
https://doi.org/10.2217/epi-2022-0214
Melman YF, Shah R, Danielson K, Xiao J, Simonson B, Barth A et al. Circulating MicroRNA-30d is associated with response to cardiac resynchronization therapy in heart failure and regulates cardiomyocyte apoptosis: a translational pilot study. Circulation. 2015;131(25):2202-16.
https://doi.org/10.1161/CIRCULATIONAHA.114.013220
Zychlinsky A, Prevost MC, Sansonetti PJ. Shigella flexneri induces apoptosis in infected macrophages. Nature. 1992;358(6382):167-9.
https://doi.org/10.1038/358167a0
Jorgensen I, Miao EA. Pyroptotic cell death defends against intracellular pathogens. Immunol Rev. 2015;265(1):130-42.
https://doi.org/10.1111/imr.12287
Bergsbaken T, Fink SL, Cookson BT. Pyroptosis: host cell death and inflammation. Nat Rev Microbiol. 2009;7(2):99-109.
https://doi.org/10.1038/nrmicro2070
Gao Y, Shi H, Dong Z, Zhang F, Sun A, Ge J. Current knowledge of pyroptosis in heart diseases. J Mol Cell Cardiol. 2022;171:81-89.
https://doi.org/10.1016/j.yjmcc.2022.07.005
Wei X, Xie F, Zhou X, Wu Y, Yan H, Liu T et al. Role of pyroptosis in inflammation and cancer. Cell Mol Immunol. 2022;19(9):971-92.
https://doi.org/10.1038/s41423-022-00905-x
Lu F, Lan Z, Xin Z, He C, Guo Z, Xia X et al. Emerging insights into molecular mechanisms underlying pyroptosis and functions of inflammasomes in diseases. J Cell Physiol. 2020;235(4):3207-21.
https://doi.org/10.1002/jcp.29268
Arakelian T, Oosterhuis K, Tondini E, Los M, Vree J, van Geldorp M et al. Pyroptosis-inducing active caspase-1 as a genetic adjuvant in anti-cancer DNA vaccination. Vaccine. 2022;40(13):2087-98.
https://doi.org/10.1016/j.vaccine.2022.02.028
Sfera A, Bullock K, Price A, Inderias L, Osorio C. Ferrosenescence: the iron age of neurodegeneration? Mech Ageing Dev. 2018;174:63-75.
https://doi.org/10.1016/j.mad.2017.11.012
Chen X, Yu C, Kang R, Tang D. Iron metabolism in ferroptosis. Front Cell Dev Biol. 2020;8:590226.
https://doi.org/10.3389/fcell.2020.590226
Kim S, Jing K, Shin S, Jeong S, Han S-H, Oh H et al. ω3-polyunsaturated fatty acids induce cell death through apoptosis and autophagy in glioblastoma cells: In vitro and in vivo. Oncol Rep. 2018;39(1):239-46.
https://doi.org/10.3892/or.2017.6101
Que X, Hung M-Y, Yeang C, Gonen A, Prohaska TA, Sun X et al. Oxidized phospholipids are proinflammatory and proatherogenic in hypercholesterolaemic mice. Nature. 2018;558(7709):301-6.
https://doi.org/10.1038/s41586-018-0198-8
Yang WS, Stockwell BR. Ferroptosis: death by lipid peroxidation. Trends Cell Biol. 2016;26(3):165-76.
https://doi.org/10.1016/j.tcb.2015.10.014
Jia C, Chen H, Zhang J, Zhou K, Zhuge Y, Niu C et al. Role of pyroptosis in cardiovascular diseases. Int Immunopharmacol. 2019;67:311-8.
https://doi.org/10.1016/j.intimp.2018.12.028
Wang Q, Wu J, Zeng Y, Chen K, Wang C, Yang S et al. Pyroptosis: a pro-inflammatory type of cell death in cardiovascular disease. Clin Chim Acta. 2020;510:62-72.
https://doi.org/10.1016/j.aca.2020.08.045
https://doi.org/10.1016/j.cca.2020.06.044
Benjamin EJ, Blaha MJ, Chiuve SE, Cushman M, Das SR, Deo R et al. Heart disease and stroke statistics-2017 update: a report from the American Heart Association. Circulation. 2017;135(10):e146-e603.
https://doi.org/10.1161/CIR.0000000000000491
Moriya J. Critical roles of inflammation in atherosclerosis. J Cardiol. 2019;73(1):22-7.
https://doi.org/10.1016/j.jjcc.2018.05.010
Xu Y-J, Zheng L, Hu Y-W, Wang Q. Pyroptosis and its relationship to atherosclerosis. Clin Chim Acta. 2018;476:28-37.
https://doi.org/10.1016/j.cca.2017.11.005
Zhaolin Z, Guohua L, Shiyuan W, Zuo W. Role of pyroptosis in cardiovascular disease. Cell Prolif. 2019;52(2):e12563.
https://doi.org/10.1111/cpr.12563
Brown PM, Kennedy DJ, Morton RE, Febbraio M. CD36/SR-B2-TLR2 dependent pathways enhance Porphyromonas gingivalis mediated atherosclerosis in the Ldlr KO mouse model. PLoS One. 2015;10(5):e0125126.
https://doi.org/10.1371/journal.pone.0125126
Wu X, Zhang H, Qi W, Zhang Y, Li J, Li Z et al. Nicotine promotes atherosclerosis via ROS-NLRP3-mediated endothelial cell pyroptosis. Cell Death Dis. 2018;9(2): 171.
https://doi.org/10.1038/s41419-017-0257-3
Chen K, Zhang J, Zhang W, Zhang J, Yang J, Li K et al. ATP-P2X4 signaling mediates NLRP3 inflammasome activation: a novel pathway of diabetic nephropathy. Int J Biochem Cell Biol. 2013;45(5):932-43.
https://doi.org/10.1016/j.biocel.2013.02.009
Luo B, Li B, Wang W, Liu X, Xia Y, Zhang C et al. NLRP3 gene silencing ameliorates diabetic cardiomyopathy in a type 2 diabetes rat model. PLoS One. 2014;9(8):e104771.
https://doi.org/10.1371/journal.pone.0104771
Li G, Xing W, Zhang M, Geng F, Yang H, Zhang H et al. Antifibrotic cardioprotection of berberine via downregulating myocardial IGF-1 receptor-regulated MMP-2/MMP-9 expression in diabetic rats. Am J Physiol Heart Circ Physiol. 2018;315(4):H802-H13.
https://doi.org/10.1152/ajpheart.00093.2018
Liu J, Kang R, Tang D. Metabolic checkpoint of ferroptosis resistance. Mol Cell Oncol. 2021;8(3):1901558.
https://doi.org/10.1080/23723556.2021.1901558
Song X, Liu J, Kuang F, Chen X, Zeh III HJ, Kang R et al. PDK4 dictates metabolic resistance to ferroptosis by suppressing pyruvate oxidation and fatty acid synthesis. Cell Rep. 2021;34(8):108767.
https://doi.org/10.1016/j.celrep.2021.108767
Cheng Z, Fang T, Huang J, Guo Y, Alam M, Qian H. Hypertrophic Cardiomyopathy From Phenotype and Pathogenesis to Treatment. Front Cardiovasc Med. 2021;8:.
https://doi.org/10.3389/fcvm.2021.722340
Li D, Pi W, Sun Z, Liu X, Jiang J. Ferroptosis and its role in cardiomyopathy. Biomed Pharmacother. 2022;153:113279.
https://doi.org/10.1016/j.biopha.2022.113279
Wu X, Li Y, Zhang S, Zhou X. Ferroptosis as a novel therapeutic target for cardiovascular disease. Theranostics. 2021;11(7):3052.
https://doi.org/10.7150/thno.54113
Yang X, Kawasaki NK, Min J, Matsui T, Wang F. Ferroptosis in heart failure. J Mol Cell Cardiol. 2022;173:141-153.
https://doi.org/10.1016/j.yjmcc.2022.10.004
Jeyabal P, Thandavarayan RA, Joladarashi D, Babu SS, Krishnamurthy S, Bhimaraj A. MicroRNA-9 inhibits hyperglycemia-induced pyroptosis in human ventricular cardiomyocytes by targeting ELAVL1. Biochem Biophys Res Commun. 2016;471(4):423-9.
https://doi.org/10.1016/j.bbrc.2016.02.065
Yang F, Qin Y, Wang Y, Li A, Lv J, Sun X et al. LncRNA KCNQ1OT1 mediates pyroptosis in diabetic cardiomyopathy. Cell Physiol Biochem. 2018;50(4):1230-44.
https://doi.org/10.1159/000494576
Zhu Y-F, Wang R, Chen W, Cao Y-D, Li L-P, Chen X. miR-133a-3p attenuates cardiomyocyte hypertrophy through inhibiting pyroptosis activation by targeting IKKε. Acta Histochem. 2021;123(1):151653.
https://doi.org/10.1016/j.acthis.2020.151653
Li X, Du N, Zhang Q, Li J, Chen X, Liu X et al. MicroRNA-30d regulates cardiomyocyte pyroptosis by directly targeting foxo3a in diabetic cardiomyopathy. Cell Death Dis. 2014;5(10):e1479-e.
https://doi.org/10.1038/cddis.2014.430
Wang Z, Xia Q, Su W, Cao M, Sun Y, Zhang M et al. Exploring the Communal Pathogenesis, Ferroptosis Mechanism, and Potential Therapeutic Targets of Dilated Cardiomyopathy and Hypertrophic Cardiomyopathy via a Microarray Data Analysis. Front Cardiovasc Med. 2022;9:824756.
https://doi.org/10.3389/fcvm.2022.824756
Gong C-W, Yuan M-M, Qiu B-Q, Wang L-J, Zou H-X, Hu T et al. Identification and Validation of Ferroptosis-Related Biomarkers in Septic Cardiomyopathy via Bioinformatics Analysis. Front Genet. 2022;13:.
https://doi.org/10.3389/fgene.2022.827559
Li X-Z, Xiong Z-C, Zhang S-L, Hao Q-Y, Gao M, Wang J-F et al. Potential ferroptosis key genes in calcific aortic valve disease. Front Cardiovasc Med. 2022;9: 916841.
https://doi.org/10.3389/fcvm.2022.916841
Zhuang Y, Yang D, Shi S, Wang L, Yu M, Meng X et al. MiR-375-3p Promotes Cardiac Fibrosis by Regulating the Ferroptosis Mediated by GPX4. Comput Intell Neurosci. 2022;2022:.
https://doi.org/10.1155/2022/9629158
Ni T, Huang X, Pan S, Lu Z. Inhibition of the long non-coding RNA ZFAS1 attenuates ferroptosis by sponging miR-150-5p and activates CCND2 against diabetic cardiomyopathy. J Cell Mol Med. 2021;25(21):9995-10007.
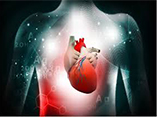
Published
How to Cite
Issue
Section
License
Copyright (c) 2023 Galen Medical Journal

This work is licensed under a Creative Commons Attribution 4.0 International License.